توربين غازي

التوربين الغازي إنگليزية: Gas turbine، ويسمى أيضاً توربين الاحتراق، هي نوع من محركات الاحتراق الداخلي ذات التدفق المستمر. تشكل الأجزاء الرئيسية المشتركة لجميع المحركات التوربينية الغازية الجزء المنتج للطاقة (المعروف باسم مولد الغاز أو القلب) وهي في اتجاه التدفق:
- ضاغط الغاز الدوار
- جهاز الاحتراق
- عنفة تعمل بالضاغط.
يجب إضافة مكونات إضافية إلى مولد الغاز لتلائم تطبيقاته. الشائع للجميع هو مدخل الهواء ولكن مع تكوينات مختلفة لتناسب متطلبات الاستخدام البحري أو استخدام الأرض أو الطيران بسرعات تتراوح من ثابت إلى أسرع من الصوت. يتم إضافة فوهة دافعة لإنتاج قوة دفع للانطلاق. تتم إضافة توربين إضافي لتشغيل مروحة (محرك مروحة عنفية) أو مروحة أنبوبية (محرك عنفي مروحي) لتقليل استهلاك الوقود (عن طريق زيادة كفاءة الدفع) بسرعات طيران دون سرعة الصوت. يلزم أيضاً وجود توربين إضافي لتشغيل دوار مروحية أو ناقل حركة أرضية (محرك عمود دوران توربيني)، أو مروحة بحرية أو مولد كهربائي (توربين طاقة). يتم تحقيق نسبة الدفع إلى الوزن الأكبر للطيران بإضافة الاحتراق المساعد.
العملية الأساسية للتوربينات الغازية هي دورة برايتون مع الهواء كسائل عامل: يتدفق الهواء الجوي عبر الضاغط الذي يرفع الضغط؛ ثم يتم إضافة الطاقة عن طريق رش الوقود في الهواء وإشعاله بحيث يولد الاحتراق تدفقاً بدرجة حرارة عالية؛ يدخل هذا الغاز المضغوط ذو درجة الحرارة العالية إلى التوربينات، مما ينتج عنه ناتج عمل رمزي في العملية، يستخدم لدفع الضاغط؛ تخرج الطاقة غير المستخدمة في غازات العادم التي يمكن إعادة توجيهها للعمل الخارجي، مثل الإنتاج المباشر للدفع في محرك نفاث عنفي، أو تدوير التوربينات المستقلة الثانية (المعروفة باسم توربينات الطاقة يمكن توصيله بمروحة أو مدسرة أو مولد كهربائي. يحدد الغرض من التوربينات الغازية التصميم بحيث يتم تحقيق أكثر تقسيم مرغوب فيه للطاقة بين الدفع وعمل العمود. تم حذف الخطوة الرابعة من دورة برايتون (تبريد السائل العامل)، حيث إن توربينات الغاز هي أنظمة مفتوحة لا تعيد استخدام نفس الهواء.
تُستخدم التوربينات الغازية لتشغيل الطائرات والقطارات والسفن والمولدات الكهربائية والمضخات وضواغط الغاز والدبابات.[1]
. . . . . . . . . . . . . . . . . . . . . . . . . . . . . . . . . . . . . . . . . . . . . . . . . . . . . . . . . . . . . . . . . . . . . . . . . . . . . . . . . . . . . . . . . . . . . . . . . . . . . . . . . . . . . . . . . . . . . . . . . . . . . . . . . . . . . . . . . . . . . . . . . . . . . . . . . . . . . . . . . . . . . . . .
الجدول الزمني للتطوير
- 50: أقدم سجلات لمحرك هيرون (كرة آيولوس). على الأرجح لم يخدم أي غرض عملي، بل كان فضولًا؛ ومع ذلك، فقد أظهر مبدأً هاماً في الفيزياء تعتمد عليه جميع المحركات التوربينية الحديثة.
- 1000: استخدم الصينيون "مصباح الحصان المهرول" (صينية: 走马灯�، zŏumădēng) في معارض الفوانيس في وقت مبكر مثل أسرة سونگ الشمالية. عندما يضيء المصباح، يرتفع تدفق الهواء الساخن ويقود دافعاً مثبتاً عليه شخصيات لركوب الخيل، ثم تُسقط ظلالها على الشاشة الخارجية للفانوس.[2]
- 1500: رُسم Smoke jack بواسطة ليوناردو دا ڤنشي: الهواء الساخن من النار يرتفع من خلال دوّار توربيني محوري أحادي المرحلة مركب في مجرى عادم الموقد ويدير حرق السيخ عن طريق توصيل سلسلة التروس.
- 1629: تقوم نفثات من البخار بتدوير توربين نبضي أدى بعد ذلك إلى تشغيل سكاكة الأختام عن طريق ترس مخطط بشكل مائل، طوره جيوڤاني برانكا.
- 1678: بنى فرديناند ڤيربيست نموذج عربة يعتمد على بخار نفاث للطاقة.
- 1791: مُنحت براءة اختراع لجون باربر، رجل إنجليزي، لأول توربين غازي حقيقي. احتوى اختراعه على معظم العناصر الموجودة في توربينات الغاز الحديثة. تم تصميم التوربين لتشغيل عربة بدون أحصنة.[3][4]
- 1861: مُنحت براءة اختراع بريطانية رقم. 1633 لمارك أنطوان فرانسوا مينونز عن "محرك حراري". تُظهر براءة الاختراع أنها كانت توربيناً غازياً وتظهر الرسومات أنه تم تطبيقه على قاطرة.[5]وقد ورد اسمه أيضاً في براءة الاختراع نيكولاس دي تيليشيف (بخلاف ذلك نيكولاس أ. تيليشوف)، وهو رائد طيران روسي.[6]
- 1872: يُعتقد أن المحرك التوربيني الغازي الذي صممه مهندس من برلين فرانز شتولتزه هو المحاولة الأولى لإنشاء نموذج عملي، لكن المحرك لم يعمل أبداً بقوته الخاصة.
- 1894: حصل السير تشارلز پارسونز على براءة اختراع لفكرة دفع سفينة باستخدام توربين بخاري، وبنى سفينة تجريبية، توربينيا، وهي أسرع سفينة طافية بسهولة في ذلك الوقت. لا يزال مبدأ الدفع هذا مفيداً بعض الشيء.
- 1895: رُكبت ثلاثة مولدات تدفق شعاعي بوزن 4 طن 100 KW پارسونز في محطة الطاقة كمبردج، واستخدمت لتشغيل أول مخطط كهربائي لإنارة الشوارع في المدينة.
- 1899: حصل تشارلز گوردون كرتس على براءة اختراع لأول محرك توربيني غازي في الولايات المتحدة ("جهاز لتوليد الطاقة الميكانيكية"، براءة اختراع رقم US635919).[7][8][9]
- 1900: قدم سانفورد ألكسندر موس أطروحة عن التوربينات الغازية. في عام 1903، أصبح موس مهندساً في قسم التوربينات البخارية التابعة لشركة جنرال إلكتريك في لين، مساتشوستس.[10] أثناء وجوده هناك، طبق بعض مفاهيمه في تطوير شاحن عنفي. استخدم تصميمه عجلة توربينية صغيرة، مدفوعة بغازات العادم، لتشغيل شاحن فائق.[10]
- 1903: قام النرويجي Ægidius Elling ببناء أول توربين غازي كان قادراً على إنتاج طاقة أكثر من اللازم لتشغيل مكوناته الخاصة، وهو ما كان يعتبر إنجازاً في وقت كانت فيه المعرفة بالديناميكا الهوائية محدودة. باستخدام الضواغط الدوارة والتوربينات، أنتجت 11 حصاناً.[11]
- 1906: المحرك التوربيني Armengaud-Lemale في فرنسا مع غرفة احتراق مبردة بالماء.
- 1910: تحقق التوربينات النبضية هولزوارث (احتراق النبض) 150 kW (200 hp).
- 1913: حصل نيكولا تيسلا على براءة اختراع لتوربين تسلا على أساس طبقة حدية.[12]
- 1920s تم تطوير النظرية العملية لتدفق الغاز عبر الممرات إلى نظرية أكثر رسمية (وقابلة للتطبيق على التوربينات) لتدفق الغاز عبر الجنيحات بواسطة الان گرفث مما أدى إلى نشر "نظرية الديناميكا الهوائية لتصميم التوربينات" في عام 1926. تم إجراء تصميمات اختبار العمل للتوربينات المحورية المناسبة لقيادة المروحة تم تطويره بواسطة المؤسسة الملكية للطيران، مما يثبت كفاءة التشكيل الديناميكي الهوائي للشفرات في عام 1929.[بحاجة لمصدر]
- 1930: حصل فرانك ويتل على براءة اختراع بعد أن لم يجد أي اهتمام بفكرته من سلاح الجو الملكي البريطاني.[13]وذلك لتصميم توربينات غازية تعمل بالطرد المركزي من أجل الدفع النفاث. حدث أول استخدام ناجح لمحركه في إنگلترا في أبريل 1937.[14]
- 1932: بدأت BBC براون، بوڤيري وسي السويسرية في بيع ضاغط محوري وتوربينات غازية كجزء من توليد البخار التوربيني غلاية ڤيلوكس. وفقاً لمبدأ التوربينات الغازية، يتم ترتيب أنابيب التبخر البخارية داخل غرفة احتراق التوربينات الغازية؛ تم إنشاء أول مصنع ڤيلوكس في موندڤيل، كالڤادو، فرنسا.[15]
- 1934: حصل راؤول پاتيراس دي پيسكارا على براءة اختراع محرك الاحتراق الحر كمولد غاز للتوربينات الغازية.[16]
- 1936: شكّل ويتل مع الآخرين المدعومين استثمار پاور جت المحدودة[بحاجة لمصدر]
- 1937: تشغيل نموذج أولي لمحرك نفاث يعمل لإثبات صحة المفهوم في المملكة المتحدة (فرانك ويتل) وألمانيا (هانس فون أوهاين Heinkel HeS 1). يؤمن هنري تيزارد تمويل الحكومة البريطانية لمزيد من التطوير لمحرك پاور جت.[17]
- 1939: أول توربين غازي لتوليد الطاقة بقدرة 4 ميگاوات من بي بي سي براون، بوڤيري آند سي لمحطة طاقة طارئة في نوشاتيل، سويسرا.[18]
- 1944: دخل محرك Junkers Jumo 004 مرحلة الإنتاج الكامل، حيث يعمل على تشغيل أولى الطائرات العسكرية الألمانية مثل مسرشميت إم إي 262. يمثل هذا بداية عهد التوربينات الغازية في السماء.
- 1946: تشكلت المؤسسة الوطنية للتوربينات الغازية من شركة پاور جتس وقسم التوربينات RAE للجمع بين عمل ويتل وهاين كونستانت.[19]في بزناو، تم تكليف أول وحدة تجارية مُعاد تسخينها/استعادتها بتوليد 27 ميگاوات.[20]
- 1947: أصبحت متروپوليتان-ڤيكرز G1 (گاتريك) أول توربين غازي بحري عندما يكمل التجارب البحرية على سفينة البحرية الملكية M.G.B 2009. كانت گاتريك عبارة عن توربين غازي مشتق من الهواء يعتمد على محرك نفاث Metropolitan Vickers F2.[21][22]
- 1995: أصبحت سيمنز أول شركة مصنعة لتوربينات الغاز الكبيرة المنتجة للكهرباء لدمج تكنولوجيا ريش التوربينات أحادية البلورة في نماذج إنتاجها، مما يسمح بدرجات حرارة تشغيل أعلى وكفاءة أكبر.[23]
- في 2011 اختبرت ميتسوبيشي للصناعات الثقيلة أول كفاءة دورة مركبة توربينات غازية (M501J) بكفاءة >60% في أعمال تاكاساگو، هيوگو.[24][25]
نظرية التشغيل
في التوربينات الغازية المثالية، تخضع الغازات لأربع عمليات ديناميكية حرارية: انضغاط متساوي الأنتروپية، احتراق متساوي الضغط (ضغط ثابت)، تمدد متساوي الانحدار ونبذ الحرارة. يشكل هؤلاء معاً دورة برايتون.
في التوربينات الغازية الحقيقية، تتغير الطاقة الميكانيكية بشكل لا رجوع فيه (بسبب الاحتكاك الداخلي والاضطراب) إلى ضغط وطاقة حرارية عند ضغط الغاز (إما في الطرد المركزي أو ضاغط الغاز) المحوري. تضاف الحرارة في غرفة الاحتراق ويزداد الحجم النوعي للغاز، مصحوباً بفقدان طفيف في الضغط. أثناء التمدد عبر ممرات الجزء الثابت والدوار في التوربين، يحدث تحول طاقة لا رجعة فيه مرة أخرى. يتم أخذ الهواء النقي بدلاً من طرد الحرارة.
إذا كان المحرك يحتوي على توربين طاقة مضافاً لتشغيل مولد صناعي أو دوار مروحية، فسيكون ضغط الخروج قريباً من ضغط الدخول قدر الإمكان مع بقاء طاقة كافية فقط للتغلب على خسائر الضغط في مجرى العادم وطرد العادم. بالنسبة لمحرك محرك مروحة عنفية، سيكون هناك توازن معين بين قوة المروحة والدفع النفاث الذي يعطي التشغيل الأكثر اقتصاداً. في محرك نفاث عنفي يتم استخراج ما يكفي من الضغط والطاقة من التدفق لدفع الضاغط والمكونات الأخرى. يتم تسريع غازات الضغط العالي المتبقية من خلال فوهة لتوفير طائرة نفاثة لدفع الطائرة.
كلما كان المحرك أصغر، يجب أن يكون معدل دوران العمود أعلى للوصول إلى سرعة طرف الشفرة المطلوبة. وتحدد سرعة طرف النصل نسب الضغط القصوى التي يمكن الحصول عليها بواسطة التوربين والضاغط. وهذا بدوره يحد من القوة القصوى والكفاءة التي يمكن أن يحصل عليها المحرك. لكي تظل سرعة الطرف ثابتة، إذا تم تقليل قطر العضو الدوار بمقدار النصف، يجب أن تتضاعف سرعة الدوران. على سبيل المثال، تعمل المحركات النفاثة الكبيرة حوالي 10000-25000 دورة في الدقيقة، بينما تدور التوربينات الصغيرة بسرعة تصل إلى 500000 دورة في الدقيقة.[26]
ميكانيكياً، "يمكن" أن تكون توربينات الغاز أقل تعقيداً من مكابس محركات الاحتراق الداخلي. قد تحتوي التوربينات البسيطة على جزء متحرك رئيسي واحد، وهو مجموعة الضاغط/العمود/دوار التوربين، مع الأجزاء المتحركة الأخرى في نظام الوقود. وهذا بدوره يمكن أن يترجم إلى سعر. على سبيل المثال، بتكلفة 10,000 للمواد، أثبت Jumo 004 أنه أرخص من محرك مكبس Junkers 213، والذي كان 35,000،[27]وتحتاج إلى 375 ساعة فقط من العمالة ذات المهارات الأقل لإكمالها (بما في ذلك التصنيع والتجميع والشحن)، مقارنة بـ 1400 ساعة BMW 801.[28] مع ذلك، شرح أيضاً إلى ضعف الكفاءة والموثوقية. قد تحتوي توربينات الغاز الأكثر تقدماً (مثل تلك الموجودة في المحركات العنفية المروحية أو محطات توليد الطاقة ذات الدورة المركبة) على 2 أو 3 أعمدة (بكرات)، ومئات من شفرات الضاغط والتوربينات، وشفرات الجزء الثابت المتحركة، وأنابيب خارجية واسعة النطاق لأنظمة الوقود والزيت والهواء؛ إنها تستخدم سبائك مقاومة للحرارة، ومصنوعة بمواصفات صارمة تتطلب تصنيعاً دقيقاً. كل هذا يجعل بناء التوربينات الغازية البسيطة أكثر تعقيداً من محرك المكبس.
علاوة على ذلك، للوصول إلى الأداء الأمثل في محطات توليد الطاقة الغازية الحديثة، يجب أن يكون الغاز مستعداً لمواصفات الوقود الدقيقة. تعالج أنظمة تكييف غاز الوقود الغاز الطبيعي للوصول إلى مواصفات الوقود الدقيقة قبل دخول التوربين من حيث الضغط ودرجة الحرارة وتكوين الغاز ومؤشر wobbe ذي الصلة.
الميزة الأساسية للمحرك التوربيني الغازي هي نسبة قوته إلى الوزن.[بحاجة لمصدر] نظراً لأنه يمكن إنشاء عمل مفيد كبير بواسطة محرك خفيف الوزن نسبياً، فإن التوربينات الغازية مناسبة تماماً لدفع الطائرات.
تعتبر محمل الدفع والمحامل البسيطة جزءاً هاماً من التصميم. إنها محامل زيت هيدروديناميكية أو مبردة بالزيت محمل ذو عناصر متدحرجة. تُستخدم المحامل الصفحية في بعض الآلات الصغيرة مثل التوربينات الصغيرة[29]ولديها أيضاً إمكانات قوية للاستخدام في توربينات الغاز الصغيرة/[[وحدة طاقة مساعدة |وحدات الطاقة المساعدة]][30]
تغير الشكل (التشوه)
يتمثل التحدي الرئيسي الذي يواجه تصميم التوربينات، وخاصة ريش التوربينات، في تقليل التشوه الناجم عن درجات الحرارة المرتفعة والضغوط التي تتعرض لها أثناء التشغيل. يتم البحث باستمرار عن درجات حرارة تشغيل أعلى من أجل زيادة الكفاءة، ولكن تأتي على حساب معدلات تشوه أعلى. لذلك تم استخدام العديد من الطرق في محاولة لتحقيق الأداء الأمثل مع الحد من التشوه، وأكثرها نجاحاً هي الطلاءات عالية الأداء وسبائك فائقة أحادية البلورة.[31]تعمل هذه التقنيات من خلال الحد من التشوه الذي يحدث من خلال آليات يمكن تصنيفها على نطاق واسع على أنها انحدار رض، صعود خلع وتدفق انتشاري.
توفر الطلاءات الواقية عزلاً حرارياً للشفرة وتوفر مقاومة للأكسدة والتآكل. غالباً ما يتم تثبيت طلاءات الحاجز الحراري (TBCs) ثنائي أكسيد الزركونيوم - الخزف القائم على الأكسدة/الطلاء المقاوم للتآكل (معاطف السندات) تتكون عادةً من الألومينيد أو سبائك (حيث يكون M هو الحديد و/أو الكروم عادةً). يؤدي استخدام TBCs إلى الحد من التعرض لدرجة الحرارة لركيزة السبائك الفائقة، وبالتالي تقليل انتشار الأنواع النشطة (عادة ما تكون الشواغر) داخل السبيكة وتقليل الانزياح وزحف الفراغ. لقد وجد أن طلاء يتراوح من 1 إلى 200 ميكرومتر يمكن أن يقلل درجة حرارة الشفرة بما يصل إلى 200 °C (392 °F).[32] يتم تطبيق أغطية مقيدة مباشرة على سطح الركيزة باستخدام كربنة العبوة وتخدم الغرض المزدوج المتمثل في توفير التصاق محسن لـ TBC ومقاومة الأكسدة للركيزة. يشكل Al من طبقات السندات Al2O3 على واجهة طبقة رابطة TBC التي توفر مقاومة الأكسدة، ولكنها تؤدي أيضاً إلى تكوين تداخل غير مرغوب فيه (ID) بينه وبين الركيزة. [33] تفوق مقاومة الأكسدة العيوب المرتبطة بمنطقة التعرف لأنها تزيد من عمر الشفرة وتحد من خسائر الكفاءة الناتجة عن التراكم على السطح الخارجي للشفرات.[34]
تتميز السبائك الفائقة القائمة على النيكل بالقوة المحسنة ومقاومة الزحف نظراً لتكوينها وبنيتها الدقيقة الناتجة. يُخلط نيكل گاما (γ) بالألمنيوم والتيتانيوم من أجل تعجيل تشتت منتظم لمراحل غاما الأولية (γ') المتماسكة Ni
3(Al,Ti). تعوق ترسبات γ' المشتتة بدقة حركة الخلع وإدخال إجهاد العتبة، مما يزيد من الضغط المطلوب لبدء التشوه. علاوة على ذلك، فإن γ' هي مرحلة مرتبة L12 تجعل من الصعب على الاضطرابات أن تتخطى ذلك.[35] يمكن إضافة المزيد من عناصر خزف حرارى مثل الرنيوم والروثنيوم في محلول صلب لتحسين مقاومة التشوه. تقلل إضافة هذه العناصر من انتشار طور گاما الأولي، وبالتالي الحفاظ على مقاومة التعب والقوة ومقاومة التشوه.[36] أدى تطوير السبائك الفائقة البلورية المفردة إلى تحسينات كبيرة في مقاومة الزحف أيضاً. بسبب عدم وجود حدود لحصول حبوب في الغطاء، تقضي البلورات المفردة على تشوه كوبل وبالتالي تتشوه بأوضاع أقل - مما يقلل من معدل الزحف.[37] على الرغم من أن البلورات المفردة لها زحف أقل في درجات الحرارة المرتفعة، إلا أنها تتمتع بضغوط إنتاجية أقل بكثير في درجة حرارة الغرفة حيث يتم تحديد القوة من خلال علاقة هول-پتش. يجب توخي الحذر من أجل تحسين پارمترات التصميم للحد من التشوه بسبب درجات الحرارة المرتفعة مع عدم تقليل قوة الخضوع في درجات الحرارة المنخفضة.
الأنواع
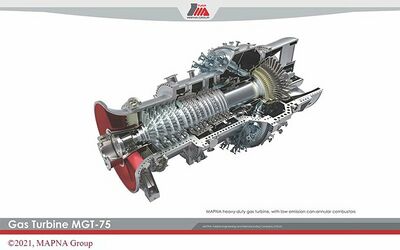
المحركات النفاثة
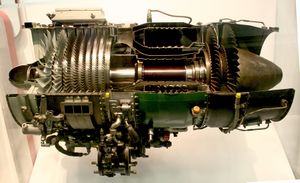
إن تنفيس الهواء المحركات النفاثة عبارة عن توربينات غازية مُحسَّنة لإنتاج قوة دفع من غازات العادم، أو من مروحة مغلفة متصلة بالتوربينات الغازية.[39]غالباً ما تسمى المحركات النفاثة التي تنتج قوة دفع من النبضات المباشرة لغازات عادم النفاث العنفي، في حين أن المحركات التي تولد الدفع مع إضافة مروحة أنبوبية تسمى غالباً محرك عنفي مروحي أو (نادراً) مروحة نفاثة .
تُستخدم توربينات الغاز أيضاً في العديد من صاروخ الوقود السائل، حيث تُستخدم توربينات الغاز لتشغيل المضخة التوربينية للسماح باستخدام خزانات خفيفة الوزن منخفضة الضغط، مما يقلل الوزن الفارغ للصاروخ.
محركات مروحية عنفية
المحرك المروحي العنفي هو محرك توربيني يقود مروحة طائرة باستخدام جهاز تخفيض السرعة. تُستخدم محركات المروحية العنفية في الطائرات الصغيرة مثل الطيران العام سيسنا 208 كاراڤان وإمبراير إي إم بي 312 توكانو وطائرات الركاب متوسطة الحجم مثل بومباردييه داش 8 والطائرات الكبيرة مثل طائرة النقل ايرباص إيه 400 إم والقاذفة الاستراتيجية البالغة من العمر 60 عاماً توپوليف تييو-95.
توربينات غازية المشتقة للطيران

تعتمد التوربينات الغازية المشتقة للطيران عموماً على محركات التوربينات الغازية للطائرات، وهي أصغر حجماً وأخف وزناً من توربينات الغاز الصناعية.[40]
تُستخدم مشتقات الطيران في توليد الطاقة الكهربائية نظراً لقدرتها على الإغلاق والتعامل مع تغيرات الأحمال بسرعة أكبر من الآلات الصناعية.[بحاجة لمصدر] كما أنها تستخدم في الصناعات البحرية لتقليل الوزن. تشمل الأنواع الشائعة جنرال إلكترك إل إم 2500 و جنرال إلكترك إل إم 6000 والإصدارات الهوائية من پرات آند ويتني پي دبليو4000 ورولز رويس أر بي 211.[40]
توربينات غازية غير احترافية
تُستخدم أعداد متزايدة من توربينات الغاز أو حتى تُبنى من قبل الهواة.
في أبسط أشكالها، هذه توربينات تجارية يتم الحصول عليها من خلال الفائض العسكري أو مبيعات ساحة الخردة، ثم يتم تشغيلها للعرض كجزء من هواية جمع المحركات.[41][42]في أشكاله المبالغ بها، قام الهواة بإعادة بناء المحركات إلى ما بعد الإصلاح الاحترافي ثم استخدموها للتنافس على الرقم القياسي لسرعة الأرض.
أبسط شكل من أشكال التوربينات الغازية ذاتية الإنشاء يستخدم شاحن عنفي كمكون أساسي. يتم تصنيع غرفة الاحتراق وتوصيلها بين قسمي الضاغط والتوربينات.[43]
كما تم بناء محركات نفاثة أكثر تطوراً، حيث يكون دفعها ووزنها الخفيف كافيين لتشغيل الطائرات ذات الطراز الكبير.[44] يقوم شركلنگ بتصميم[44] وبناء المحرك بالكامل من المواد الخام، بما في ذلك تصنيع عجلة ضاغط طرد مركزي من الخشب الرقائقي، والإيپوكسي وخيوط ألياف الكربون الملفوفة.
تقوم العديد من الشركات الصغيرة الآن بتصنيع توربينات وأجزاء صغيرة للهواة. تستخدم معظم الطائرات النموذجية التي تعمل بالطاقة النفاثة الآن هذه التوربينات الصغيرة التجارية وشبه التجارية، بدلاً من التصميمات المنزلية الشبيهة بشركلنگ.[45]
. . . . . . . . . . . . . . . . . . . . . . . . . . . . . . . . . . . . . . . . . . . . . . . . . . . . . . . . . . . . . . . . . . . . . . . . . . . . . . . . . . . . . . . . . . . . . . . . . . . . . . . . . . . . . . . . . . . . . . . . . . . . . . . . . . . . . . . . . . . . . . . . . . . . . . . . . . . . . . . . . . . . . . . .
وحدات الطاقة المساعدة
تُستخدم توربينات الغاز الصغيرة كوحدات طاقة مساعدة (APUs) لتزويد الطاقة المساعدة للآلات الأكبر حجماً والمتحركة مثل المركبات الفضائية. يزودون:
- هواء مضغوط للتكييف والتهوية،
- طاقة بدء تشغيل الهواء المضغوط من أجل محركات نفاثة أكبر،
- القدرة الميكانيكية (العمود) إلى علبة التروس لتشغيل الملحقات المحورية أو لبدء تشغيل المحركات النفاثة الكبيرة، و
- المصادر الكهربائية والهيدروليكية وغيرها من مصادر نقل الطاقة إلى الأجهزة المستهلكة البعيدة عن وحدة APU.
Industrial gas turbines for power generation


تختلف توربينات الغاز الصناعي عن تصميمات الطيران من حيث أن الهياكل والمحامل والشفرات ثقيلة البناء. كما أنها أكثر تكاملاً مع الأجهزة التي تشغلها - غالباً المولدات الكهربائية - ومعدات الطاقة الثانوية التي تُستخدم لاستعادة الطاقة المتبقية (الحرارة إلى حد كبير).
وتتراوح أحجامها من محطات متنقلة محمولة إلى أنظمة كبيرة ومعقدة تزن أكثر من مائة طن في مبانٍ مشيدة لهذا الغرض. عندما يتم استخدام التوربينات الغازية فقط من أجل الطاقة المحورية، فإن كفاءتها الحرارية تكون حوالي 30٪. ومع ذلك، قد يكون شراء الكهرباء أرخص من توليدها. لذلك، يتم استخدام العديد من المحركات في تكوينات CHP (الحرارة والطاقة المجمعة) التي يمكن أن تكون صغيرة بما يكفي لدمجها في تكوينات الحاوية المحمولة.
يمكن أن تكون توربينات الغاز فعالة بشكل خاص عندما يتم استرداد الحرارة المهدرة من التوربين بواسطة مولد بخار لاسترداد الحرارة (HRSG) لتشغيل توربينات بخارية تقليدية في دورة مركبة.[46] حقق المولد 605 ميگاواط جنرال إلكتريك 9HA معدل كفاءة بنسبة 62.22٪ مع درجات حرارة عالية تصل إلى 1,540 °C (2,800 °F).[47] بالنسبة لعام 2018، تقدم جنرال إلكتريك قدرة 826 ميگاواط من HA بكفاءة تزيد عن 64٪ في الدورة المركبة بسبب التقدم في التصنيع الإضافي واختراقات الاحتراق، ارتفاعاً من 63.7٪ في طلبات 2017 وعلى المسار الصحيح لتحقيق 65٪ بحلول أوائل عام 2020.[48] في مارس 2018، حققت GE Power كفاءة إجمالية قدرها 63.08٪ لتوربينها 7HA.[49]
يمكن أيضاً استخدام توربينات الغاز المشتقة من الهواء في الدورات المركبة، مما يؤدي إلى كفاءة أعلى، ولكنها لن تكون عالية مثل التوربينات الغازية الصناعية المصممة خصيصاً. يمكن أيضاً تشغيلها في تكوين التوليد المشترك للطاقة: يتم استخدام العادم في الفضاء أو تسخين المياه، أو تشغيل مبرد الامتصاص لتبريد الهواء الداخل وزيادة خرج الطاقة، وهي تقنية تُعرف باسم توربين مدخل تبريد الهواء.
ميزة أخرى مهمة هي قدرتها على التشغيل والإيقاف في غضون دقائق، وتوفير الطاقة أثناء الذروة، أو الطلب غير المجدول. نظراً لأن محطات توليد الطاقة ذات الدورة الواحدة (توربينات الغاز فقط) أقل كفاءة من محطات الدورة المركبة، فإنها تُستخدم عادةً كـ محطة توليد الذروة، والتي تعمل في أي مكان من عدة ساعات يومياً إلى بضع عشرات من الساعات سنوياً - اعتماداً على الطلب على الكهرباء والقدرة التوليدية للمنطقة. في المناطق التي تعاني من نقص في الحمل الأساسي والقدرة الحمل التالي لمحطة توليد الطاقة أو بتكاليف وقود منخفضة، قد تعمل محطة توليد الطاقة التوربينية الغازية بانتظام معظم ساعات اليوم. ينتج التوربينات الغازية الكبيرة أحادية الدورة عادةً 100 إلى 400 ميگاواط من الطاقة الكهربائية وتحتوي على 35-40٪ من الكفاءة الديناميكية الحرارية.[50]
التوربينات الغازية الصناعية للقيادة الميكانيكية
تختلف التوربينات الغازية الصناعية التي تُستخدم فقط للمحرك الميكانيكي أو تُستخدم بالتعاون مع مولد بخار الاسترداد عن مجموعات توليد الطاقة من حيث أنها غالباً ما تكون أصغر حجماً وتتميز بتصميم عمود مزدوج بدلاً من عمود واحد. يتراوح نطاق الطاقة من 1 ميگاواط إلى 50 ميگاواط.[بحاجة لمصدر] هذه المحركات متصلة مباشرة أو عبر علبة تروس إما بمضخة أو ضاغط. تستخدم غالبية المنشآت في صناعات النفط والغاز. تزيد تطبيقات المحرك الميكانيكي من الكفاءة بحوالي 2٪.
تتطلب منصات النفط والغاز هذه المحركات لدفع الضواغط لحقن الغاز في الآبار لدفع النفط عبر حفرة أخرى، أو لضغط الغاز للنقل. وغالباً ما تُستخدم أيضاً لتوفير الطاقة للمنصة. لا تحتاج هذه المنصات إلى استخدام المحرك بالتعاون مع نظام CHP نظراً للحصول على الغاز بتكلفة منخفضة للغاية (غالباً خالية من حرق الغاز). تستخدم نفس الشركات مجموعات المضخات لدفع السوائل إلى الأرض وعبر خطوط الأنابيب في فترات زمنية مختلفة.
تخزين طاقة الهواء المضغوط
يسعى أحد التطورات الحديثة إلى تحسين الكفاءة بطريقة أخرى، عن طريق فصل الضاغط عن التوربين بمخزن هواء مضغوط. في التوربينات التقليدية، يتم استخدام ما يصل إلى نصف الطاقة المولدة لتشغيل الضاغط. في تكوين تخزين طاقة الهواء المضغوط، يتم استخدام الطاقة، ربما من مزرعة الرياح أو شراؤها من السوق المفتوحة في وقت انخفاض الطلب والسعر المنخفض، لتشغيل الضاغط، ويتم إطلاق الهواء المضغوط لتشغيل التوربين عند الحاجة.
محركات عمود الدوران التوربيني
تستخدم محركات عمود الدوران التوربيني لتشغيل الضواغط في محطات ضخ الغاز ومحطات تسييل الغاز الطبيعي. كما أنها تستخدم لتشغيل جميع طائرات الهليكوبتر الحديثة باستثناء أصغرها. العمود الأساسي يحمل الضاغط والتوربينات الخاصة به والتي تسمى مع جهاز الاحتراق "مولد الغاز". عادة ما يتم استخدام التوربينات الكهربائية التي تدور بشكل منفصل لقيادة الدوار على طائرات الهليكوبتر. يسمح لمولد الغاز وتوربينات الطاقة/الدوار بالدوران بسرعاتهما الخاصة مما يتيح مزيداً من المرونة في تصميمهما.
التوربينات الغازية القطرية
المحركات النفاثة الصغيرة
تُعرف أيضاً باسم التوربينات الغازية المصغرة أو الطائرات النفاثة الصغيرة.
مع وضع هذا في الاعتبار، أنتج رائد مايكرو-جتس الحديثة، كرت شركلنگ، واحدة من أولى التوربينات الصغيرة في العالم،FD3/67.[44]يمكن لهذا المحرك إنتاج ما يصل إلى 22 نيوتن من قوة الدفع، ويمكن تصنيعه بواسطة معظم الأشخاص ذوي التفكير الميكانيكي باستخدام الأدوات الهندسية الأساسية، مثل المخرطة المعدنية.[44]
التوربينات الدقيقة
تطورت التوربينات الدقيقة من شواحن توربينية ذات مكبس متحرك، APU للطائرات أو محركات نفاثة صغيرة، من 25 إلى 500 كيلو واط من توربينات بحجم ثلاجة. تمتلك التوربينات الدقيقة حوالي 15٪ الكفاءة بدون استرجاع، 20 إلى 30٪ مع واحد ويمكن أن تصل إلى 85٪ من الكفاءة الحرارية والكهربائية المجمعة في التوليد المشترك للطاقة.[51]
الاحتراق الخارجي
معظم التوربينات الغازية هي محركات احتراق داخلي ولكن من الممكن أيضاً تصنيع توربين غاز احتراق خارجي وهو، بشكل فعال، نسخة توربينية من محرك هواء ساخن. يشار إلى هذه الأنظمة عادةً باسم EFGT (توربينات غازية تعمل خارجياً) أو IFGT (توربينات غازية تعمل بشكل غير مباشر).
تم استخدام الاحتراق الخارجي لغرض استخدام الفحم المسحوق أو الكتلة الحيوية المطحونة بدقة (مثل نشارة الخشب) كوقود. في النظام غير المباشر، يتم استخدام مبادل حراري وينتقل الهواء النظيف الذي لا يحتوي على منتجات احتراق عبر توربينات الطاقة. تكون الكفاءة الحرارية أقل في النوع غير المباشر من الاحتراق الخارجي؛ ومع ذلك، فإن شفرات التوربينات لا تخضع لمنتجات الاحتراق ويمكن استخدام أنواع وقود أقل جودة (وبالتالي أرخص).
عند استخدام الاحتراق الخارجي، يمكن استخدام هواء العادم من التوربين باعتباره هواء الاحتراق الأساسي. هذا يقلل بشكل فعال من خسائر الحرارة العالمية، على الرغم من أن فقدان الحرارة المرتبط بعادم الاحتراق لا يزال حتمياً.
تبشر التوربينات الغازية ذات الدورة المغلقة التي تعتمد على الهليوم أو ثنائي أكسيد الكربون فوق الحرج أيضاً باستخدامها مع توليد الطاقة الشمسية والنووية ذات درجات الحرارة العالية في المستقبل.
. . . . . . . . . . . . . . . . . . . . . . . . . . . . . . . . . . . . . . . . . . . . . . . . . . . . . . . . . . . . . . . . . . . . . . . . . . . . . . . . . . . . . . . . . . . . . . . . . . . . . . . . . . . . . . . . . . . . . . . . . . . . . . . . . . . . . . . . . . . . . . . . . . . . . . . . . . . . . . . . . . . . . . . .
In surface vehicles
Gas turbines are often used on ships, locomotives, helicopters, tanks, and to a lesser extent, on cars, buses, and motorcycles.
A key advantage of jets and turboprops for airplane propulsion – their superior performance at high altitude compared to piston engines, particularly naturally aspirated ones – is irrelevant in most automobile applications. Their power-to-weight advantage, though less critical than for aircraft, is still important.
Gas turbines offer a high-powered engine in a very small and light package. However, they are not as responsive and efficient as small piston engines over the wide range of RPMs and powers needed in vehicle applications. In series hybrid vehicles, as the driving electric motors are mechanically detached from the electricity generating engine, the responsiveness, poor performance at low speed and low efficiency at low output problems are much less important. The turbine can be run at optimum speed for its power output, and batteries and ultracapacitors can supply power as needed, with the engine cycled on and off to run it only at high efficiency. The emergence of the continuously variable transmission may also alleviate the responsiveness problem.
Turbines have historically been more expensive to produce than piston engines, though this is partly because piston engines have been mass-produced in huge quantities for decades, while small gas turbine engines are rarities; however, turbines are mass-produced in the closely related form of the turbocharger.
The turbocharger is basically a compact and simple free shaft radial gas turbine which is driven by the piston engine's exhaust gas. The centripetal turbine wheel drives a centrifugal compressor wheel through a common rotating shaft. This wheel supercharges the engine air intake to a degree that can be controlled by means of a wastegate or by dynamically modifying the turbine housing's geometry (as in a variable geometry turbocharger). It mainly serves as a power recovery device which converts a great deal of otherwise wasted thermal and kinetic energy into engine boost.
Turbo-compound engines (actually employed on some semi-trailer trucks) are fitted with blow down turbines which are similar in design and appearance to a turbocharger except for the turbine shaft being mechanically or hydraulically connected to the engine's crankshaft instead of to a centrifugal compressor, thus providing additional power instead of boost. While the turbocharger is a pressure turbine, a power recovery turbine is a velocity one.
Passenger road vehicles (cars, bikes, and buses)
A number of experiments have been conducted with gas turbine powered automobiles, the largest by Chrysler.[52][53] More recently, there has been some interest in the use of turbine engines for hybrid electric cars. For instance, a consortium led by micro gas turbine company Bladon Jets has secured investment from the Technology Strategy Board to develop an Ultra Lightweight Range Extender (ULRE) for next-generation electric vehicles. The objective of the consortium, which includes luxury car maker Jaguar Land Rover and leading electrical machine company SR Drives, is to produce the world's first commercially viable – and environmentally friendly – gas turbine generator designed specifically for automotive applications.[54]
The common turbocharger for gasoline or diesel engines is also a turbine derivative.
Concept cars
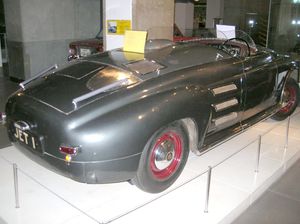
The first serious investigation of using a gas turbine in cars was in 1946 when two engineers, Robert Kafka and Robert Engerstein of Carney Associates, a New York engineering firm, came up with the concept where a unique compact turbine engine design would provide power for a rear wheel drive car. After an article appeared in Popular Science, there was no further work, beyond the paper stage.[55]
In 1950, designer F.R. Bell and Chief Engineer Maurice Wilks from British car manufacturers Rover unveiled the first car powered with a gas turbine engine. The two-seater JET1 had the engine positioned behind the seats, air intake grilles on either side of the car, and exhaust outlets on the top of the tail. During tests, the car reached top speeds of 140 km/h (87 mph), at a turbine speed of 50,000 rpm. After being shown in the United Kingdom and the United States in 1950, JET1 was further developed, and was subjected to speed trials on the Jabbeke highway in Belgium in June 1952, where it exceeded 240 km/h (150 mph).[56] The car ran on petrol, paraffin (kerosene) or diesel oil, but fuel consumption problems proved insurmountable for a production car. JET1 is on display at the London Science Museum.
A French turbine-powered car, the SOCEMA-Grégoire, was displayed at the October 1952 Paris Auto Show. It was designed by the French engineer Jean-Albert Grégoire.[57]
The first turbine-powered car built in the US was the GM Firebird I which began evaluations in 1953. While photos of the Firebird I may suggest that the jet turbine's thrust propelled the car like an aircraft, the turbine actually drove the rear wheels. The Firebird 1 was never meant as a commercial passenger car and was solely built for testing & evaluation as well as public relation purposes.[58]
Starting in 1954 with a modified Plymouth,[59] the American car manufacturer Chrysler demonstrated several prototype gas turbine-powered cars from the early 1950s through the early 1980s. Chrysler built fifty Chrysler Turbine Cars in 1963 and conducted the only consumer trial of gas turbine-powered cars.[60] Each of their turbines employed a unique rotating recuperator, referred to as a regenerator that increased efficiency.[59]
In 1954 Fiat unveiled a concept car with a turbine engine, called Fiat Turbina. This vehicle, looking like an aircraft with wheels, used a unique combination of both jet thrust and the engine driving the wheels. Speeds of 282 km/h (175 mph) were claimed.[61]
The original General Motors Firebird was a series of concept cars developed for the 1953, 1956 and 1959 Motorama auto shows, powered by gas turbines.
In 1960's, Ford and GM were developing gas turbine semi-trucks. One such concept truck was known as the Big Red. With the trailer, it was 29 m (96 ft) long and 4.0 m (13 ft) high and painted crimson red. It contained the Ford developed gas turbine engine, with 450 kW (600 hp) and 1,160 N⋅m (855 lb⋅ft). The cab boasted a highway map of the continental U.S., a mini-kitchen, bathroom, and a TV for the co-driver. The fate of the truck was unknown for several decades, but it was rediscovered in early 2021 in private hands, having been restored to running order.[62][63][64]
As a result of the U.S. Clean Air Act Amendments of 1970, research was funded into developing automotive gas turbine technology.[65] Design concepts and vehicles were conducted by Chrysler, General Motors, Ford (in collaboration with AiResearch), and American Motors (in conjunction with Williams Research).[66] Long-term tests were conducted to evaluate comparable cost efficiency.[67] Several AMC Hornets were powered by a small Williams regenerative gas turbine weighing 250 lb (113 kg) and producing 80 hp (60 kW; 81 PS) at 4450 rpm.[68][69][70]
In 1982, General Motors used an Oldsmobile Delta 88 powered by a gas turbine using pulverised coal dust. This was considered for the United States and the western world to reduce dependence on middle east oil at the time[71][72][73]
Toyota demonstrated several gas turbine powered concept cars, such as the Century gas turbine hybrid in 1975, the Sports 800 Gas Turbine Hybrid in 1979 and the GTV in 1985. No production vehicles were made. The GT24 engine was exhibited in 1977 without a vehicle.
In the early 1990s, Volvo introduced the Volvo ECC which was a gas turbine powered hybrid electric vehicle.[74]
In 1993 General Motors introduced the first commercial gas turbine powered hybrid vehicle—as a limited production run of the EV-1 series hybrid. A Williams International 40 kW turbine drove an alternator which powered the battery-electric powertrain. The turbine design included a recuperator. In 2006, GM went into the EcoJet concept car project with Jay Leno.
At the 2010 Paris Motor Show Jaguar demonstrated its Jaguar C-X75 concept car. This electrically powered supercar has a top speed of 204 mph (328 km/h) and can go from 0 to 62 mph (0 to 100 km/h) in 3.4 seconds. It uses Lithium-ion batteries to power four electric motors which combine to produce 780 bhp. It will travel 68 miles (109 km) on a single charge of the batteries, and uses a pair of Bladon Micro Gas Turbines to re-charge the batteries extending the range to 560 miles (900 km).[75]
Racing cars


The first race car (in concept only) fitted with a turbine was in 1955 by a US Air Force group as a hobby project with a turbine loaned them by Boeing and a race car owned by Firestone Tire & Rubber company.[76] The first race car fitted with a turbine for the goal of actual racing was by Rover and the BRM Formula One team joined forces to produce the Rover-BRM, a gas turbine powered coupe, which entered the 1963 24 Hours of Le Mans, driven by Graham Hill and Richie Ginther. It averaged 107.8 mph (173.5 km/h) and had a top speed of 142 mph (229 km/h). American Ray Heppenstall joined Howmet Corporation and McKee Engineering together to develop their own gas turbine sports car in 1968, the Howmet TX, which ran several American and European events, including two wins, and also participated in the 1968 24 Hours of Le Mans. The cars used Continental gas turbines, which eventually set six FIA land speed records for turbine-powered cars.[77]
For open wheel racing, 1967's revolutionary STP-Paxton Turbocar fielded by racing and entrepreneurial legend Andy Granatelli and driven by Parnelli Jones nearly won the Indianapolis 500; the Pratt & Whitney ST6B-62 powered turbine car was almost a lap ahead of the second place car when a gearbox bearing failed just three laps from the finish line. The next year the STP Lotus 56 turbine car won the Indianapolis 500 pole position even though new rules restricted the air intake dramatically. In 1971 Team Lotus principal Colin Chapman introduced the Lotus 56B F1 car, powered by a Pratt & Whitney STN 6/76 gas turbine. Chapman had a reputation of building radical championship-winning cars, but had to abandon the project because there were too many problems with turbo lag.
Buses
The arrival of the Capstone Turbine has led to several hybrid bus designs, starting with HEV-1 by AVS of Chattanooga, Tennessee in 1999, and closely followed by Ebus and ISE Research in California, and DesignLine Corporation in New Zealand (and later the United States). AVS turbine hybrids were plagued with reliability and quality control problems, resulting in liquidation of AVS in 2003. The most successful design by Designline is now operated in 5 cities in 6 countries, with over 30 buses in operation worldwide, and order for several hundred being delivered to Baltimore, and New York City.
Brescia Italy is using serial hybrid buses powered by microturbines on routes through the historical sections of the city.[78]
Motorcycles
The MTT Turbine Superbike appeared in 2000 (hence the designation of Y2K Superbike by MTT) and is the first production motorcycle powered by a turbine engine – specifically, a Rolls-Royce Allison model 250 turboshaft engine, producing about 283 kW (380 bhp). Speed-tested to 365 km/h or 227 mph (according to some stories, the testing team ran out of road during the test), it holds the Guinness World Record for most powerful production motorcycle and most expensive production motorcycle, with a price tag of US$185,000.
Trains
Several locomotive classes have been powered by gas turbines, the most recent incarnation being Bombardier's JetTrain.
Tanks
The Third Reich Wehrmacht Heer's development division, the Heereswaffenamt (Army Ordnance Board), studied a number of gas turbine engine designs for use in tanks starting in mid-1944. The first gas turbine engine design intended for use in armored fighting vehicle propulsion, the BMW 003-based GT 101, was meant for installation in the Panther tank.[79]
The second use of a gas turbine in an armored fighting vehicle was in 1954 when a unit, PU2979, specifically developed for tanks by C. A. Parsons and Company, was installed and trialed in a British Conqueror tank.[80] The Stridsvagn 103 was developed in the 1950s and was the first mass-produced main battle tank to use a turbine engine, the Boeing T50. Since then, gas turbine engines have been used as auxiliary power units in some tanks and as main powerplants in Soviet/Russian T-80s and U.S. M1 Abrams tanks, among others. They are lighter and smaller than diesel engines at the same sustained power output but the models installed to date are less fuel efficient than the equivalent diesel, especially at idle, requiring more fuel to achieve the same combat range. Successive models of M1 have addressed this problem with battery packs or secondary generators to power the tank's systems while stationary, saving fuel by reducing the need to idle the main turbine. T-80s can mount three large external fuel drums to extend their range. Russia has stopped production of the T-80 in favor of the diesel-powered T-90 (based on the T-72), while Ukraine has developed the diesel-powered T-80UD and T-84 with nearly the power of the gas-turbine tank. The French Leclerc tank's diesel powerplant features the "Hyperbar" hybrid supercharging system, where the engine's turbocharger is completely replaced with a small gas turbine which also works as an assisted diesel exhaust turbocharger, enabling engine RPM-independent boost level control and a higher peak boost pressure to be reached (than with ordinary turbochargers). This system allows a smaller displacement and lighter engine to be used as the tank's power plant and effectively removes turbo lag. This special gas turbine/turbocharger can also work independently from the main engine as an ordinary APU.
A turbine is theoretically more reliable and easier to maintain than a piston engine since it has a simpler construction with fewer moving parts, but in practice, turbine parts experience a higher wear rate due to their higher working speeds. The turbine blades are highly sensitive to dust and fine sand so that in desert operations air filters have to be fitted and changed several times daily. An improperly fitted filter, or a bullet or shell fragment that punctures the filter, can damage the engine. Piston engines (especially if turbocharged) also need well-maintained filters, but they are more resilient if the filter does fail.
Like most modern diesel engines used in tanks, gas turbines are usually multi-fuel engines.
Marine applications
Gas turbines are used in many naval vessels, where they are valued for their high power-to-weight ratio and their ships' resulting acceleration and ability to get underway quickly.
The first gas-turbine-powered naval vessel was the Royal Navy's Motor Gun Boat MGB 2009 (formerly MGB 509) converted in 1947. Metropolitan-Vickers fitted their F2/3 jet engine with a power turbine. The Steam Gun Boat Grey Goose was converted to Rolls-Royce gas turbines in 1952 and operated as such from 1953.[81] The Bold class Fast Patrol Boats Bold Pioneer and Bold Pathfinder built in 1953 were the first ships created specifically for gas turbine propulsion.[82]
The first large-scale, partially gas-turbine powered ships were the Royal Navy's Type 81 (Tribal class) frigates with combined steam and gas powerplants. The first, إتشإمإس Ashanti was commissioned in 1961.
The German Navy launched the first Köln-طراز frigate in 1961 with 2 Brown, Boveri & Cie gas turbines in the world's first combined diesel and gas propulsion system.
The Soviet Navy commissioned in 1962 the first of 25 Kashin-طراز destroyer with 4 gas turbines in Combined gas and gas propulsion system. Those vessels used 4 M8E gas turbines, which generated 54,000–72,000 kW (72,000–96,000 hp). Those ships were the first large ships in the world to be powered solely by gas turbines.
The Danish Navy had 6 Søløven-class torpedo boats (the export version of the British Brave class fast patrol boat) in service from 1965 to 1990, which had 3 Bristol Proteus (later RR Proteus) Marine Gas Turbines rated at 9,510 kW (12,750 shp) combined, plus two General Motors Diesel engines, rated at 340 kW (460 shp), for better fuel economy at slower speeds.[83] And they also produced 10 Willemoes Class Torpedo / Guided Missile boats (in service from 1974 to 2000) which had 3 Rolls Royce Marine Proteus Gas Turbines also rated at 9,510 kW (12,750 shp), same as the Søløven-class boats, and 2 General Motors Diesel Engines, rated at 600 kW (800 shp), also for improved fuel economy at slow speeds.[84]
The Swedish Navy produced 6 Spica-class torpedo boats between 1966 and 1967 powered by 3 Bristol Siddeley Proteus 1282 turbines, each delivering 3,210 kW (4,300 shp). They were later joined by 12 upgraded Norrköping class ships, still with the same engines. With their aft torpedo tubes replaced by antishipping missiles they served as missile boats until the last was retired in 2005.[85]
The Finnish Navy commissioned two Turunmaa، طراز corvettes, Turunmaa and Karjala, in 1968. They were equipped with one 16,410 kW (22,000 shp) Rolls-Royce Olympus TM1 gas turbine and three Wärtsilä marine diesels for slower speeds. They were the fastest vessels in the Finnish Navy; they regularly achieved speeds of 35 knots, and 37.3 knots during sea trials. The Turunmaas were decommissioned in 2002. Karjala is today a museum ship in Turku, and Turunmaa serves as a floating machine shop and training ship for Satakunta Polytechnical College.
The next series of major naval vessels were the four Canadian Iroquois، طراز helicopter carrying destroyers first commissioned in 1972. They used 2 ft-4 main propulsion engines, 2 ft-12 cruise engines and 3 Solar Saturn 750 kW generators.

The first U.S. gas-turbine powered ship was the U.S. Coast Guard's Point Thatcher, a cutter commissioned in 1961 that was powered by two 750 kW (1,000 shp) turbines utilizing controllable-pitch propellers.[86] The larger Hamilton، طراز High Endurance Cutters, was the first class of larger cutters to utilize gas turbines, the first of which (USCGC Hamilton) was commissioned in 1967. Since then, they have powered the U.S. Navy's Oliver Hazard Perry، طراز frigates, Spruance and Arleigh Burke، طراز destroyers, and Ticonderoga، طراز guided missile cruisers. يوإسإس Makin Island, a modified Wasp-طراز amphibious assault ship, is to be the Navy's first amphibious assault ship powered by gas turbines. The marine gas turbine operates in a more corrosive atmosphere due to the presence of sea salt in air and fuel and use of cheaper fuels.
Civilian maritime
Up to the late 1940s, much of the progress on marine gas turbines all over the world took place in design offices and engine builder's workshops and development work was led by the British Royal Navy and other Navies. While interest in the gas turbine for marine purposes, both naval and mercantile, continued to increase, the lack of availability of the results of operating experience on early gas turbine projects limited the number of new ventures on seagoing commercial vessels being embarked upon.
In 1951, the Diesel-electric oil tanker Auris, 12,290 deadweight tonnage (DWT) was used to obtain operating experience with a main propulsion gas turbine under service conditions at sea and so became the first ocean-going merchant ship to be powered by a gas turbine. Built by Hawthorn Leslie at Hebburn-on-Tyne, UK, in accordance with plans and specifications drawn up by the Anglo-Saxon Petroleum Company and launched on the UK's Princess Elizabeth's 21st birthday in 1947, the ship was designed with an engine room layout that would allow for the experimental use of heavy fuel in one of its high-speed engines, as well as the future substitution of one of its diesel engines by a gas turbine.[87] The Auris operated commercially as a tanker for three-and-a-half years with a diesel-electric propulsion unit as originally commissioned, but in 1951 one of its four 824 kW (1,105 bhp) diesel engines – which were known as "Faith", "Hope", "Charity" and "Prudence" – was replaced by the world's first marine gas turbine engine, a 890 kW (1,200 bhp) open-cycle gas turbo-alternator built by British Thompson-Houston Company in Rugby. Following successful sea trials off the Northumbrian coast, the Auris set sail from Hebburn-on-Tyne in October 1951 bound for Port Arthur in the US and then Curacao in the southern Caribbean returning to Avonmouth after 44 days at sea, successfully completing her historic trans-Atlantic crossing. During this time at sea the gas turbine burnt diesel fuel and operated without an involuntary stop or mechanical difficulty of any kind. She subsequently visited Swansea, Hull, Rotterdam, Oslo and Southampton covering a total of 13,211 nautical miles. The Auris then had all of its power plants replaced with a 3,910 kW (5,250 shp) directly coupled gas turbine to become the first civilian ship to operate solely on gas turbine power.
Despite the success of this early experimental voyage the gas turbine did not replace the diesel engine as the propulsion plant for large merchant ships. At constant cruising speeds the diesel engine simply had no peer in the vital area of fuel economy. The gas turbine did have more success in Royal Navy ships and the other naval fleets of the world where sudden and rapid changes of speed are required by warships in action.[88]
The United States Maritime Commission were looking for options to update WWII Liberty ships, and heavy-duty gas turbines were one of those selected. In 1956 the John Sergeant was lengthened and equipped with a General Electric 4,900 kW (6,600 shp) HD gas turbine with exhaust-gas regeneration, reduction gearing and a variable-pitch propeller. It operated for 9,700 hours using residual fuel (Bunker C) for 7,000 hours. Fuel efficiency was on a par with steam propulsion at 0.318 kg/kW (0.523 lb/hp) per hour,[89] and power output was higher than expected at 5,603 kW (7,514 shp) due to the ambient temperature of the North Sea route being lower than the design temperature of the gas turbine. This gave the ship a speed capability of 18 knots, up from 11 knots with the original power plant, and well in excess of the 15 knot targeted. The ship made its first transatlantic crossing with an average speed of 16.8 knots, in spite of some rough weather along the way. Suitable Bunker C fuel was only available at limited ports because the quality of the fuel was of a critical nature. The fuel oil also had to be treated on board to reduce contaminants and this was a labor-intensive process that was not suitable for automation at the time. Ultimately, the variable-pitch propeller, which was of a new and untested design, ended the trial, as three consecutive annual inspections revealed stress-cracking. This did not reflect poorly on the marine-propulsion gas-turbine concept though, and the trial was a success overall. The success of this trial opened the way for more development by GE on the use of HD gas turbines for marine use with heavy fuels.[90] The John Sergeant was scrapped in 1972 at Portsmouth PA.

Boeing launched its first passenger-carrying waterjet-propelled hydrofoil Boeing 929, in April 1974. Those ships were powered by two Allison 501-KF gas turbines.[91]
Between 1971 and 1981, Seatrain Lines operated a scheduled container service between ports on the eastern seaboard of the United States and ports in northwest Europe across the North Atlantic with four container ships of 26,000 tonnes DWT. Those ships were powered by twin Pratt & Whitney gas turbines of the FT 4 series. The four ships in the class were named Euroliner, Eurofreighter, Asialiner and Asiafreighter. Following the dramatic Organization of the Petroleum Exporting Countries (OPEC) price increases of the mid-1970s, operations were constrained by rising fuel costs. Some modification of the engine systems on those ships was undertaken to permit the burning of a lower grade of fuel (i.e., marine diesel). Reduction of fuel costs was successful using a different untested fuel in a marine gas turbine but maintenance costs increased with the fuel change. After 1981 the ships were sold and refitted with, what at the time, was more economical diesel-fueled engines but the increased engine size reduced cargo space.[بحاجة لمصدر]
The first passenger ferry to use a gas turbine was the GTS Finnjet, built in 1977 and powered by two Pratt & Whitney FT 4C-1 DLF turbines, generating 55,000 kW (74,000 shp) and propelling the ship to a speed of 31 knots. However, the Finnjet also illustrated the shortcomings of gas turbine propulsion in commercial craft, as high fuel prices made operating her unprofitable. After four years of service, additional diesel engines were installed on the ship to reduce running costs during the off-season. The Finnjet was also the first ship with a Combined diesel-electric and gas propulsion. Another example of commercial use of gas turbines in a passenger ship is Stena Line's HSS class fastcraft ferries. HSS 1500-class Stena Explorer, Stena Voyager and Stena Discovery vessels use combined gas and gas setups of twin GE LM2500 plus GE LM1600 power for a total of 68,000 kW (91,000 shp). The slightly smaller HSS 900-class Stena Carisma, uses twin ABB–STAL GT35 turbines rated at 34,000 kW (46,000 shp) gross. The Stena Discovery was withdrawn from service in 2007, another victim of too high fuel costs.[بحاجة لمصدر]
In July 2000 the Millennium became the first cruise ship to be powered by both gas and steam turbines. The ship featured two General Electric LM2500 gas turbine generators whose exhaust heat was used to operate a steam turbine generator in a COGES (combined gas electric and steam) configuration. Propulsion was provided by two electrically driven Rolls-Royce Mermaid azimuth pods. The liner RMS Queen Mary 2 uses a combined diesel and gas configuration.[92]
In marine racing applications the 2010 C5000 Mystic catamaran Miss GEICO uses two Lycoming T-55 turbines for its power system.[بحاجة لمصدر]
Advances in technology
Gas turbine technology has steadily advanced since its inception and continues to evolve. Development is actively producing both smaller gas turbines and more powerful and efficient engines. Aiding in these advances are computer-based design (specifically computational fluid dynamics and finite element analysis) and the development of advanced materials: Base materials with superior high-temperature strength (e.g., single-crystal superalloys that exhibit yield strength anomaly) or thermal barrier coatings that protect the structural material from ever-higher temperatures. These advances allow higher compression ratios and turbine inlet temperatures, more efficient combustion and better cooling of engine parts.
Computational fluid dynamics (CFD) has contributed to substantial improvements in the performance and efficiency of gas turbine engine components through enhanced understanding of the complex viscous flow and heat transfer phenomena involved. For this reason, CFD is one of the key computational tools used in design and development of gas[93][94] turbine engines.
The simple-cycle efficiencies of early gas turbines were practically doubled by incorporating inter-cooling, regeneration (or recuperation), and reheating. These improvements, of course, come at the expense of increased initial and operation costs, and they cannot be justified unless the decrease in fuel costs offsets the increase in other costs. The relatively low fuel prices, the general desire in the industry to minimize installation costs, and the tremendous increase in the simple-cycle efficiency to about 40 percent left little desire for opting for these modifications.[95]
On the emissions side, the challenge is to increase turbine inlet temperatures while at the same time reducing peak flame temperature in order to achieve lower NOx emissions and meet the latest emission regulations. In May 2011, Mitsubishi Heavy Industries achieved a turbine inlet temperature of 1,600 °C on a 320 megawatt gas turbine, and 460 MW in gas turbine combined-cycle power generation applications in which gross thermal efficiency exceeds 60%.[96]
Compliant foil bearings were commercially introduced to gas turbines in the 1990s. These can withstand over a hundred thousand start/stop cycles and have eliminated the need for an oil system. The application of microelectronics and power switching technology have enabled the development of commercially viable electricity generation by microturbines for distribution and vehicle propulsion.
Advantages and disadvantages
The following are advantages and disadvantages of gas-turbine engines:[97]
Advantages include:
- Very high power-to-weight ratio compared to reciprocating engines.
- Smaller than most reciprocating engines of the same power rating.
- Smooth rotation of the main shaft produces far less vibration than a reciprocating engine.
- Fewer moving parts than reciprocating engines results in lower maintenance cost and higher reliability/availability over its service life.
- Greater reliability, particularly in applications where sustained high power output is required.
- Waste heat is dissipated almost entirely in the exhaust. This results in a high-temperature exhaust stream that is very usable for boiling water in a combined cycle, or for cogeneration.
- Lower peak combustion pressures than reciprocating engines in general.
- High shaft speeds in smaller "free turbine units", although larger gas turbines employed in power generation operate at synchronous speeds.
- Low lubricating oil cost and consumption.
- Can run on a wide variety of fuels.
- Very low toxic emissions of CO and HC due to excess air, complete combustion and no "quench" of the flame on cold surfaces.
Disadvantages include:
- Core engine costs can be high due to use of exotic materials.
- Less efficient than reciprocating engines at idle speed.
- Longer startup than reciprocating engines.
- Less responsive to changes in power demand compared with reciprocating engines.
- Characteristic whine can be hard to suppress.
Major manufacturers
- Siemens
- Ansaldo
- Mitsubishi Heavy
- Rolls Royce
- General Electric
- Silmash
- ODK
- Pratt & Whitney
- P&W Canada
- Alstom
- Zorya-Mashproekt
- MTU Aero Engines
- MAN Turbo
- IHI Corporation
- Kawasaki Heavy
- HAL
- BHEL
- MAPNA
- Techwin
- Doosan Heavy
- Shanghai Electric
- Harbin Electric
- AECC
السوق العالمية
تعرضت روسيا وكندا وألمانيا لانحدار بالسوق فيما يتعلق بالعديد من توربينات الغاز الروسية التي تم إرسالها إلى كندا للإصلاح وبدلاً من ذلك تم حظرها عندما كانت جاهزة لإعادة شحنها. حيث تبحث روسيا الآن عن شركاء جدد لتزويد هذه المعدات المتطورة.
وستقوم ألمانيا ببيع الأشياء عالية التقنية إلى دول أخرى وتمكينها.
فهي نفس التوربينات. التي قامت شركة سيمنز بنقل التكنولوجيا لبناءها في عام 2016.[98]
Testing
British, German, other national and international test codes are used to standardize the procedures and definitions used to test gas turbines. Selection of the test code to be used is an agreement between the purchaser and the manufacturer, and has some significance to the design of the turbine and associated systems. In the United States, ASME has produced several performance test codes on gas turbines. This includes ASME PTC 22–2014. These ASME performance test codes have gained international recognition and acceptance for testing gas turbines. The single most important and differentiating characteristic of ASME performance test codes, including PTC 22, is that the test uncertainty of the measurement indicates the quality of the test and is not to be used as a commercial tolerance.
See also
![]() |
- Air-start system
- Axial compressor
- Centrifugal compressor
- Distributed generation
- Gas turbine-electric locomotive
- Gas turbine locomotive
- Gas turbine modular helium reactor
- Non-intrusive stress measurement system
- Pneumatic motor
- Steam turbine
- Turbine engine failure
- Wind turbine
References
- ^ Sonntag, Richard E.; Borgnakke, Claus (2006). Introduction to engineering thermodynamics (Second ed.). John Wiley. ISBN 9780471737599.
- ^ B. Zhang (14 ديسمبر 2014). Lu, Yongxiang (ed.). A History of Chinese Science and Technology: Volume 3. Springer Berlin Heidelberg. pp. 308–310. ISBN 978-3662441626.
'For trotting horse lamp, make paper-cut as wheel-like objects and the candle will heat the air which will rise and push the paper-cut to move, and the shadows of paper-cut will be cast by the candle light on the screen.' ...Judgment from the records of the Song dynasty shows that invention of China's trotting horse lamp was not later than 1000 AD. ...Obviously, the trotting horse lamp has already had the rudiment of a gas turbine.
- ^ "Massachusetts Institute of Technology Gas Turbine Lab". Web.mit.edu. 27 أغسطس 1939. Retrieved 13 أغسطس 2012.
- ^ UK patent no. 1833 – Obtaining and Applying Motive Power, & c. A Method of Rising Inflammable Air for the Purposes of Procuring Motion, and Facilitating Metallurgical Operations
- ^ "Original document: GB186101633 (A) ― 1861-12-18 caloric engines". Worldwide.espacenet.com. Retrieved 13 مارس 2016.
- ^ Giges, Nancy (يوليو 2013). "Igor Sikorsky Aviation Pioneer". ASME. Retrieved 7 يونيو 2019.
- ^ "Patent US0635919" (PDF). Freepatentsonline.com. Retrieved 13 أغسطس 2012.
- ^ "History – Biographies, Landmarks, Patents". ASME. 10 مارس 1905. Retrieved 13 أغسطس 2012.
- ^ {{{1}}} patent {{{2}}}
- ^ أ ب Leyes, p.231-232.
- ^ Bakken, Lars E et al., p.83-88. "Centenary of the First Gas Turbine to Give Net Power Output: A Tribute to Ægidius Elling". ASME. 2004
- ^ U.S. Patent US1٬061٬206
- ^ "Welcome to the Frank Whittle Website". www.frankwhittle.co.uk. Archived from the original on 13 فبراير 2012. Retrieved 22 أكتوبر 2016.
- ^ Kreith, Frank, ed. (1998). The CRC Handbook of Mechanical Engineering (Second ed.). US: CRC Press. p. 222. ISBN 978-0-8493-9418-8.
- ^ "University of Bochum "In Touch Magazine 2005", p. 5" (PDF). Archived from the original (PDF) on 13 مارس 2012. Retrieved 13 أغسطس 2012.
- ^ Automotive News (in الإنجليزية). Crain Automotive Group. 1981.
- ^ John Golley. 1996. "Jet: Frank Whittle and the invention of the jet engine". ISBN 978-1-907472-00-8
- ^ Eckardt, D. and Rufli, P. "Advanced Gas Turbine Technology – ABB/ BBC Historical Firsts", ASME J. Eng. Gas Turb. Power, 2002, p. 124, 542–549
- ^ Giffard, Hermione (10 أكتوبر 2016). Making Jet Engines in World War II: Britain, Germany, and the United States (in الإنجليزية). University of Chicago Press. ISBN 978-0-226-38859-5.
- ^ Eckardt, D. "Gas Turbine Powerhouse". 2014. ISBN 978-3-11-035962-6
- ^ "Post War Advances in Propulsion". The Times. 15 يونيو 1953. p. 20. Retrieved 8 يناير 2021.
- ^ Nunn, Robert H (25 February 1977). The Marine Gas Turbine-The UK Provides a Case Study in Technological Development. US Office of Naval Research. pp. 5. Archived from the original. You must specify the date the archive was made using the
|archivedate=
parameter. https://apps.dtic.mil/dtic/tr/fulltext/u2/a037686.pdf. - ^ Langston, Lee S. (6 فبراير 2017). "Each Blade a Single Crystal". American Scientist. Retrieved 25 يناير 2019.
- ^ Hada, Satoshi; et al. "Test Results of the World's First 1,600C J-series Gas Turbine" (PDF). Archived from the original (PDF) on 16 أكتوبر 2015. Retrieved 15 أكتوبر 2015.
- ^ "Gas Turbines breaking the 60% efficiency barrier". Cogeneration & On-Site Power Production. 5 يناير 2010. Archived from the original on 30 سبتمبر 2013.
- ^ (2006) "Rotordynamic behaviour of a micro-turbine rotor on air bearings: modelling techniques and experimental verification, p. 182" in ISMA., International Conference on Noise and Vibration Engineering.
- ^ Christopher, John. The Race for Hitler's X-Planes (The Mill, Gloucestershire: History Press, 2013), p.74.
- ^ Christopher, p.75.
- ^ Nalepa, Krzysztof; Pietkiewicz2, Paweł; Żywica, Grzegorz (نوفمبر 2009). "Development of the foil bearing technology" (PDF). Technical Sciences. 12: 229–240. doi:10.2478/v10022-009-0019-2 (inactive 1 مارس 2022). S2CID 44838086. Retrieved 1 مارس 2022.
{{cite journal}}
: CS1 maint: DOI inactive as of 2022 (link) CS1 maint: numeric names: authors list (link) - ^ Agrawal, Giri L. (2 June 1997). "Foil Air/Gas Bearing Technology – An Overview" in ASME 1997 International Gas Turbine and Aeroengine Congress and Exhibition.: V001T04A006. doi:10.1115/97-GT-347.
- ^ (2008) "Superalloys 2008 (Eleventh International Symposium)" in Superalloys.: 753–760, US: The Minerals, Metals & Materials Society. doi:10.7449/2008/Superalloys_2008_753_760.
- ^ "Coatings for turbine blades"
- ^ A. W. James et al. "Gas turbines: operating conditions, components and material requirements"
- ^ Tamarin, Y. Protective Coatings for Turbine Blades. 2002. ASM International. pp 3–5
- ^ A. Nowotnik "Nickel-Based Superalloys"
- ^ Latief, F. H.; Kakehi, K. (2013) "Effects of Re content and crystallographic orientation on creep behavior of aluminized Ni-based single crystal superalloys". Materials & Design 49 : 485–492
- ^ Caron P., Khan T. "Evolution of Ni-based superalloys for single crystal gas turbine blade applications"
- ^ Saeed (30 يوليو 2022). "Russia will use Iranian MAPNA turbines to produce electricity instead of German Siemens". twitter.com/Haman_Ten.
- ^ Dick, Erik (2015). "Thrust Gas Turbines". Fundamentals of Turbomachines. 109.
- ^ أ ب Robb, Drew (1 ديسمبر 2017). "Aeroderivative gas turbines". Turbomachinery International Magazine. Retrieved 26 يونيو 2020.
- ^ "Vulcan APU startup". Archived from the original (video) on 13 أبريل 2013.
- ^ "Bristol Siddeley Proteus". Internal Fire Museum of Power. 1999. Archived from the original on 18 يناير 2009.
- ^ "Jet Racer". Scrapheap Challenge.
- ^ أ ب ت ث Schreckling, Kurt (1994). Gas Turbines for Model Aircraft. ISBN 978-0-9510589-1-6.
- ^ Kamps, Thomas (2005). Model Jet Engines. Traplet Publications. ISBN 978-1-900371-91-9.
- ^ Lee S. Langston (يوليو 2012). "Efficiency by the Numbers". Archived from the original on 7 فبراير 2013.
- ^ Kellner, Tomas (17 يونيو 2016). "Here's Why The Latest Guinness World Record Will Keep France Lit Up Long After Soccer Fans Leave" (Press release). General Electric.
- ^ "HA technology now available at industry-first 64 percent efficiency" (Press release). GE Power. 4 ديسمبر 2017.
- ^ "GE's HA Gas Turbine Delivers Second World Record for Efficiency" (Press release). GE Power. 27 مارس 2018.
- ^ Ratliff, Phil; Garbett, Paul; Fischer, Willibald (سبتمبر 2007). "The New Siemens Gas Turbine SGT5-8000H for More Customer Benefit" (PDF). VGB PowerTech. Siemens Power Generation. Archived from the original (PDF) on 13 أغسطس 2011. Retrieved 17 يوليو 2010.
- ^ Capehart, Barney L. (22 ديسمبر 2016). "Microturbines". Whole Building Design Guide. National Institute of Building Sciences.
- ^ "History of Chrysler Corporation Gas Turbine Vehicles" published by the Engineering Section 1979
- ^ "Chrysler Corp., Exner Concept Cars 1940 to 1961" undated, retrieved on 11 May 2008.
- ^ Bladon Jets And Jaguar Land Rover Win Funding For Gas Turbine Electric Vehicle Project Archived 13 مارس 2012 at the Wayback Machine
- ^ "Gas Turbines For Autos". Popular Science. 146 (8): 121. مايو 1946. Retrieved 13 مارس 2016.
- ^ Bobbitt, Malcolm (2007) [1994]. "III – Gas-Turbines and the Jet Era". Rover P4 Series (revised ed.). Dorchester, UK: Veloce Publishing. pp. 84–87. ISBN 978-1-903706-57-2. Retrieved 17 أكتوبر 2014.
- ^ Depreux, Stephane (فبراير 2005). "Rétromobile 2005". Classics.com. Archived from the original on 16 ديسمبر 2018.
- ^ "Gas Turbine Auto". Popular Mechanics. 101 (3): 90. مارس 1954.
- ^ أ ب "Turbo Plymouth Threatens Future of Standard". Popular Science. 165 (1): 102. يوليو 1954. Retrieved 13 مارس 2016.
- ^ "Chrysler turbine engines and cars". Allpar.com. Retrieved 13 مارس 2016.
- ^ "Italy's Turbo Car Hits 175 m.p.h." Popular Mechanics. 165 (1): 120. يوليو 1954. Retrieved 13 مارس 2016.
- ^ " Big Red " Experimental Gas Turbine Semi Truck 1964 New York World's Fair XD10344. Ford Motor Company. 1966. Archived from the original on 30 أكتوبر 2021. Retrieved 4 سبتمبر 2020 – via YouTube.
- ^ Holderith, Peter (19 أغسطس 2020). "Ford's Giant Turbine Semi-Truck 'Big Red' Is Lost Somewhere in the American Southeast". The Drive. US. Retrieved 21 أغسطس 2020.
- ^ Holderith, Peter (24 مارس 2021). "We Found Ford's Incredible Turbine-Powered Semi-Truck 'Big Red' That's Been Lost for Decades". The Drive. US. Retrieved 27 مارس 2021.
- ^ Linden, Lawrence H.; Kumar, Subramanyam; Samuelson, Paul R. (ديسمبر 1977). Issues in Federally Supported Research on Advanced Automotive Power Systems. Division of Policy Research and Analysis, National Science Foundation. p. 49. hdl:1721.1/31259.
- ^ Linden, page 53.
- ^ Verrelli, L. D.; Andary, C. J. (مايو 1972). "Exhaust Emission Analysis of the Williams Research Gas Turbine AMC Hornet". National Technical Information Service. OSTI 5038506. PB218687.
- ^ Norbye, Jan P. (مارس 1971). "Tiny 80-HP gas turbine to power compact car". Popular Science. 198 (3): 34. Retrieved 13 مارس 2016.
- ^ Ludvigsen, Karl (نوفمبر 1971). "Williams Turbine Takes the Road". Motor Trend. 23 (11).
- ^ Norbye, Jan P.; Dunne, Jim (سبتمبر 1973). "Gas turbine car: it's now or never". Popular Science. 302 (3): 59.
- ^ Roy, Rex (2 يناير 2009). "Coal in Your Stocking? Fuel up the Cadillac!". The New York Times.
- ^ "This Oldsmobile was powered by a coal-burning turbine engine". 16 يناير 2017.
- ^ "GM made a coal-powered car in the 80s".
- ^ "Article in Green Car". Greencar.com. 31 أكتوبر 2007. Archived from the original on 13 أغسطس 2012. Retrieved 13 أغسطس 2012.
- ^ Nagy, Chris (1 أكتوبر 2010). "The Electric Cat: Jaguar C-X75 Concept Supercar". Automoblog.net. Retrieved 13 مارس 2016.
- ^ "Turbine Drives Retired Racing Car". Popular Science: 89. يونيو 1955. Retrieved 23 يوليو 2018.
- ^ "The history of the Howmet TX turbine car of 1968, still the world's only turbine powered race winner". Pete Stowe Motorsport History. يونيو 2006. Archived from the original on 2 مارس 2008. Retrieved 31 يناير 2008.
- ^ "Serial Hybrid Busses for a Public Transport scheme in Brescia (Italy)". Draft.fgm-amor.at. Archived from the original on 16 مارس 2012. Retrieved 13 أغسطس 2012.
- ^ Kay, Antony L. (2002). German jet engine and gas turbine development 1930 – 1945. Airlife. ISBN 9781840372946.
- ^ Ogorkiewicz, Richard M. (1991). Technology of Tanks. Jane's Information Group. p. 259. ISBN 9780710605955.
- ^ Walsh, Philip P.; Paul Fletcher (2004). Gas Turbine Performance (2nd ed.). John Wiley and Sons. p. 25. ISBN 978-0-632-06434-2.
- ^ "The first marine gas turbine, 1947". Scienceandsociety.co.uk. 23 أبريل 2008. Retrieved 13 أغسطس 2012.
- ^ Søløven class torpedoboat, 1965 Archived 15 نوفمبر 2011 at the Wayback Machine
- ^ Willemoes class torpedo/guided missile boat, 1974 Archived 20 أغسطس 2011 at the Wayback Machine
- ^ Fast missile boat
- ^ "US Coast Guard Historian's website, USCGC Point Thatcher (WPB-82314)" (PDF). Retrieved 13 أغسطس 2012.
- ^ "Operation of a Marine Gas Turbine Under Sea Conditions". Journal of the American Society for Naval Engineers. 66 (2): 457–466. 2009. doi:10.1111/j.1559-3584.1954.tb03976.x.
- ^ Future Ship Powering Options: Exploring alternative methods of ship propulsion. Royal Academy of Engineering Prince Philip House. 2013. ISBN 9781909327016.
- ^ Naval Education and Training Program Development Center Introduction to Marine Gas Turbines (1978) Naval Education and Training Support Command, pp. 3.
- ^ National Research Council (U.S.) Innovation in the Maritime Industry (1979) Maritime Transportation Research Board, pp. 127–131
- ^ "Jetfoil/hydrofoil Historical Snapshot". Boeing.
- ^ "GE – Aviation: GE Goes from Installation to Optimized Reliability for Cruise Ship Gas Turbine Installations". Geae.com. 16 مارس 2004. Archived from the original on 16 أبريل 2011. Retrieved 13 أغسطس 2012.
- ^ "CFD for Aero Engines" (PDF). HCL Technologies. أبريل 2011. Archived from the original (PDF) on 9 يوليو 2017. Retrieved 13 مارس 2016.
- ^ Chrystie, R; Burns, I; Kaminski, C (2013). "Temperature Response of an Acoustically Forced Turbulent Lean Premixed Flame: A Quantitative Experimental Determination". Combustion Science and Technology. 185: 180–199. doi:10.1080/00102202.2012.714020. S2CID 46039754.
- ^ Çengel, Yunus A.; Boles., Michael A. (2011). 9-8. Thermodynamics: An Engineering Approach (7th ed.). New York: McGraw-Hill. p. 510.
- ^ "MHI Achieves 1,600 °C Turbine Inlet Temperature in Test Operation of World's Highest Thermal Efficiency "J-Series" Gas Turbine". Mitsubishi Heavy Industries. 26 مايو 2011. Archived from the original on 13 نوفمبر 2013.
- ^ Brain, Marshall (1 أبريل 2000). "How Gas Turbine Engines Work". Science.howstuffworks.com. Retrieved 13 مارس 2016.
- ^ Haman_Ten (30 يوليو 2022). "Russia will use Iranian MAPNA turbines to produce electricity instead of German Siemens". twitter.com/Haman_Ten.
Further reading
- Stationary Combustion Gas Turbines including Oil & Over-Speed Control System description
- "Aircraft Gas Turbine Technology" by Irwin E. Treager, McGraw-Hill, Glencoe Division, 1979, ISBN 0-07-065158-2.
- "Gas Turbine Theory" by H.I.H. Saravanamuttoo, G.F.C. Rogers and H. Cohen, Pearson Education, 2001, 5th ed., ISBN 0-13-015847-X.
- Leyes II, Richard A.; William A. Fleming (1999). The History of North American Small Gas Turbine Aircraft Engines. Washington, DC: Smithsonian Institution. ISBN 978-1-56347-332-6.
- R. M. "Fred" Klaass and Christopher DellaCorte, "The Quest for Oil-Free Gas Turbine Engines," SAE Technical Papers, No. 2006-01-3055, available at sae.org
- "Model Jet Engines" by Thomas Kamps ISBN 0-9510589-9-1 Traplet Publications
- Aircraft Engines and Gas Turbines, Second Edition by Jack L. Kerrebrock, The MIT Press, 1992, ISBN 0-262-11162-4.
- "Forensic Investigation of a Gas Turbine Event" by John Molloy, M&M Engineering
- "Gas Turbine Performance, 2nd Edition" by Philip Walsh and Paul Fletcher, Wiley-Blackwell, 2004 ISBN 978-0-632-06434-2
- ((National Academies of Sciences, Engineering, and Medicine)) (2020). 'Advanced Technologies for Gas Turbines'. Washington, DC: The National Academies Press. doi: . ISBN 978-0-309-66422-6.
External links
- توربين غازي at Curlie
- Bonnier Corporation (ديسمبر 1939). "New Era In Power To Turn Wheels". Popular Science. Bonnier Corporation. p. 81.
- Technology Speed of Civil Jet Engines
- MIT Gas Turbine Laboratory Archived 21 يوليو 2010 at the Wayback Machine
- MIT Microturbine research
- California Distributed Energy Resource guide – Microturbine generators
- Introduction to how a gas turbine works from "how stuff works.com" Archived 16 يونيو 2008 at the Wayback Machine
- Aircraft gas turbine simulator for interactive learning
- An online handbook on stationary gas turbine technologies compiled by the US DOE.
- CS1: Julian–Gregorian uncertainty
- CS1 maint: DOI inactive as of 2022
- CS1 maint: numeric names: authors list
- Short description is different from Wikidata
- Articles containing إنگليزية-language text
- Pages using Lang-xx templates
- مقالات تحتوي نصوصاً باللغة الصينية
- Articles with unsourced statements from December 2012
- Articles with unsourced statements from January 2019
- Articles with unsourced statements from June 2020
- Articles with hatnote templates targeting a nonexistent page
- Articles with Curlie links
- Use dmy dates from August 2019
- عنفات غازية
- Engines
- Marine propulsion
- تكنولوجيا محطات الطاقة
- اختراعات نرويجية