صاروخ بدافع هجين
صاروخ بدافع هجين hybrid-propellant rocket، is a rocket with a rocket motor that uses rocket propellants in two different phases: one solid and the other either gas or liquid. The hybrid rocket concept can be traced back to at least the 1930s.[1]
Hybrid rockets avoid some of the disadvantages of solid rockets like the dangers of propellant handling, while also avoiding some disadvantages of liquid rockets like their mechanical complexity.[2] Because it is difficult for the fuel and oxidizer to be mixed intimately (being different states of matter), hybrid rockets tend to fail more benignly than liquids or solids. Like liquid rocket engines, hybrid rocket motors can be shut down easily and the thrust is throttleable. The theoretical specific impulse () performance of hybrids is generally higher than solid motors and lower than liquid engines. as high as 400 s has been measured in a hybrid rocket using metalized fuels.[3] Hybrid systems are more complex than solid ones, but they avoid significant hazards of manufacturing, shipping and handling solid rocket motors by storing the oxidizer and the fuel separately.
. . . . . . . . . . . . . . . . . . . . . . . . . . . . . . . . . . . . . . . . . . . . . . . . . . . . . . . . . . . . . . . . . . . . . . . . . . . . . . . . . . . . . . . . . . . . . . . . . . . . . . . . . . . . . . . . . . . . . . . . . . . . . . . . . . . . . . . . . . . . . . . . . . . . . . . . . . . . . . . . . . . . . . . .
التاريخ
The first work on hybrid rockets was performed in the late 1930s at IG Farben in Germany and concurrently at the California Rocket Society in the United States. Leonid Andrussow, working in Germany, first theorized hybrid propellant rockets. O. Lutz, W. Noeggerath, and Andrussow tested a 10 kilonewtons (2,200 lbf) hybrid rocket motor using coal and gaseous N2O as the propellants. Oberth also worked on a hybrid rocket motor using LOX as the oxidizer and graphite as the fuel. The high heat of sublimation of carbon prevented these rocket motors from operating efficiently, as it resulted in a negligible burning rate.[4]
In the 1940s, the California Pacific Rocket Society used LOX in combination with several different fuel types, including wood, wax, and rubber. The most successful of these tests was with the rubber fuel, which is still the dominant fuel in use today. In June 1951, a LOX / rubber rocket was flown to an altitude of 9 kilometres (5.6 mi).[4]
Two major efforts occurred in the 1950s. One of these efforts was by G. Moore and K. Berman at General Electric. The duo used 90% high test peroxide (HTP, or H2O2) and polyethylene (PE) in a rod and tube grain design. They drew several significant conclusions from their work. The fuel grain had uniform burning. Grain cracks did not affect combustion, like it does with solid rocket motors. No hard starts were observed (a hard start is a pressure spike seen close to the time of ignition, typical of liquid rocket engines). The fuel surface acted as a flame holder, which encouraged stable combustion. The oxidizer could be throttled with one valve, and a high oxidizer to fuel ratio helped simplify combustion. The negative observations were low burning rates and that the thermal instability of peroxide was problematic for safety reasons. Another effort that occurred in the 1950s was development of a reverse hybrid. In a standard hybrid rocket motor, the solid material is the fuel. In a reverse hybrid rocket motor, the oxidizer is solid. William Avery of the Applied Physics Laboratory used jet fuel and ammonium nitrate, selected for their low cost. His O/F ratio was 0.035, which was 200 times smaller than the ratio used by Moore and Berman.[4]
In 1953 Pacific Rocket Society (est. 1943) was developing the XDF-23, a 4 inches (10 cm) × 72 inches (180 cm) hybrid rocket, designed by Jim Nuding, using LOX and rubber polymer called "Thiokol". They had already tried other fuels in prior iterations including cotton, paraffin wax and wood. The XDF name itself comes from "experimental Douglas fir" from one of the first units.[5]
In the 1960s, European organizations also began work on hybrid rockets. ONERA, based in France, and Volvo Flygmotor, based in Sweden, developed sounding rockets using hybrid rocket motor technology. The ONERA group focused on a hypergolic rocket motor, using nitric acid and an amine fuel. The company flew eight rockets: Once in April 1964, three times in June 1965, and four times in 1967. The maximum altitude the flights achieved was over 100 kilometres (62 mi).[4] The Volvo Flygmotor group also used a hypergolic propellant combination. They also used nitric acid for their oxidizer, but used Tagaform (polybutadiene with an aromatic amine) as their fuel. Their flight was in 1969, lofting a 20 kilograms (44 lb) payload to 80 kilometres (50 mi).[4]
Meanwhile, in the United States, United Technologies Center (Chemical Systems Division) and Beech Aircraft were working on a supersonic target drone, known as Sandpiper. It used MON-25 (mixed 25% NO, 75% N2O4) as the oxidizer and polymethyl methacrylate (PMM) and Mg for the fuel. The drone flew six times in 1968, for more than 300 seconds and to an altitude greater than 160 kilometres (99 mi). The second iteration of the rocket, known as the HAST, had IRFNA-PB/PMM for its propellants and was throttleable over a 10/1 range. HAST could carry a heavier payload than the Sandpiper. Another iteration, which used the same propellant combination as the HAST, was developed by Chemical Systems Division and Teledyne Aircraft. Development for this program ended in the mid-1980s. Chemical Systems Division also worked on a propellant combination of lithium and FLOx (mixed F2 and O2). This was an efficient hypergolic rocket that was throtteable. The vacuum specific impulse was 380 seconds at 93% combustion efficiency.[4]
AMROC developed the largest hybrid rockets ever created in the late 1980s and early 1990s. The first version of their engine, fired at the Air Force Phillips Laboratory, produced 312,000 newtons (70,000 lbf) of thrust for 70 seconds with a propellant combination of LOX and hydroxyl-terminated polybutadiene (HTPB) rubber. The second version of the motor, known as the H-250F, produced more than 1,000,000 newtons (220,000 lbf) of thrust.[4]
Korey Kline of Environmental Aeroscience Corporation (eAc) first fired a gaseous oxygen and rubber hybrid in 1982 at Lucerne Dry Lake, CA, after discussions on the technology with Bill Wood, formerly with Westinghouse.[6] The first SpaceShipOne hybrid tests were successfully conducted by Kline and eAc at Mojave, CA.[7]
In 1994, the U.S. Air Force Academy flew a hybrid sounding rocket to an altitude of 5 kilometres (3.1 mi). The 6.4 metres (21 ft) rocket used HTPB and LOX for its propellant, and reached a peak thrust of 4,400 newtons (990 lbf) and had a thrust duration of 16 seconds.[4]
مفاهيم أساسية
In its simplest form a hybrid rocket consists of a pressure vessel (tank) containing the liquid oxidiser, the combustion chamber containing the solid propellant, and a mechanical device separating the two. When thrust is desired, a suitable ignition source is introduced in the combustion chamber and the valve is opened. The liquid oxidiser (or gas) flows into the combustion chamber where it is vaporized and then reacted with the solid propellant. Combustion occurs in a boundary layer diffusion flame adjacent to the surface of the solid propellant.
Generally the liquid propellant is the oxidizer and the solid propellant is the fuel because solid oxidizers are extremely dangerous and lower performing than liquid oxidizers. Furthermore, using a solid fuel such as Hydroxyl-terminated polybutadiene (HTPB) or paraffin wax allows for the incorporation of high-energy fuel additives such as aluminium, lithium, or metal hydrides.
الإحتراق
The governing equation for hybrid rocket combustion shows that the regression rate is dependent on the oxidizer mass flux rate, which means the rate that the fuel will burn is proportional to the amount of oxidizer flowing through the port. This differs from a solid rocket motor, in which the regression rate is proportional to the chamber pressure of the motor.[4]
- where is the regression rate, ao is the regression rate coefficient (incorporating the grain length), Go is the oxidizer mass flux rate, and n is the regression rate exponent.[4]
As the motor burns, the increase in diameter of the fuel port results in an increased fuel mass flow rate. This phenomenon makes the oxidizer to fuel ratio (O/F) shift during the burn. The increased fuel mass flow rate can be compensated for by also increasing the oxidizer mass flow rate. In addition to the O/F varying as a function of time, it also varies based on the position down the fuel grain. The closer the position is to the top of the fuel grain, the higher the O/F ratio. Since the O/F varies down the port, a point called the stoichiometric point may exist at some point down the grain.[4]
الخصائص
Hybrid rocket motors exhibit some obvious as well as some subtle advantages over liquid-fuel rockets and solid-fuel rockets. A brief summary of some of these is given below:
المزايا مقارنة بصواريخ الوقود السائل
- Mechanically simpler – requires only a single liquid propellant resulting in less plumbing, fewer valves, and simpler operations.
- Denser fuel – fuels in the solid phase generally have higher density than those in the liquid phase, reducing overall system volume.
- Metal additives – reactive metals such as aluminium, magnesium, lithium or beryllium can be easily included in the fuel grain increasing specific impulse (), density, or both.
- Combustion instabilities – Hybrid rockets do not typically exhibit high frequency combustion instabilities that plague liquid rockets due to the solid fuel grain breaking up acoustic waves that would otherwise reflect in an open liquid engine combustion chamber.
- Propellant pressurization – One of the most difficult to design portions of a liquid rocket system are the turbopumps. Turbopump design is complex as it has to precisely and efficiently pump and keep separated two fluids of different properties in precise ratios at very high volumetric flow rates, often cryogenic temperatures, and highly volatile chemicals while combusting those same fluids in order to power itself. Hybrids have far less fluid to move and can often be pressurized by a blow-down system (which would be prohibitively heavy in a liquid rocket) or self-pressurized oxidizers (such as N2O).
- Cooling – Liquid rockets often depend on one of the propellants, typically the fuel, to cool the combustion chamber and nozzle due to the very high heat fluxes and vulnerability of the metal walls to oxidation and stress cracking. Hybrid rockets have combustion chambers that are lined with the solid propellant which shields it from the product gases. Their nozzles are often graphite or coated in ablative materials similarly to solid rocket motors. The design, construction, and testing of liquid cooling flows is complex, making the system more prone to failure.
المزايا مقارنة بصواريخ الوقود الصلب
- Higher theoretical – Possible due to limits of known solid oxidizers compared to often used liquid oxidizers.
- Less explosion hazard – Propellant grain is more tolerant of processing errors such as cracks since the burn rate is dependent on oxidizer mass flux rate. Propellant grain cannot be ignited by stray electrical charge and is very insensitive to auto-igniting due to heat. Hybrid rocket motors can be transported to the launch site with the oxidizer and fuel stored separately, improving safety.
- Fewer handling and storage issues – Ingredients in solid rockets are often incompatible chemically and thermally. Repeated changes in temperature can cause distortion of the grain. Antioxidants and coatings are used to keep the grain from breaking down or decomposing.
- More controllable – Stop/restart and throttling are all easily incorporated into most designs. Solid rockets rarely can be shut down easily and almost never have throttling or restart capabilities.
عيوب الصواريخ الهجين
Hybrid rockets also exhibit some disadvantages when compared with liquid and solid rockets. These include:
- Oxidizer-to-fuel ratio shift ("O/F shift") – with a constant oxidizer flow-rate, the ratio of fuel production rate to oxidizer flow rate will change as a grain regresses. This leads to off-peak operation from a chemical performance point of view. However, for a well-designed hybrid, O/F shift has a very small impact on performance because is insensitive to O/F shift near the peak.
- Low regression-rate (rate at which the solid phase recedes) fuels often drive multi-port fuel grains. Multi-port fuel grains have poor volumetric efficiency and, often, structural deficiencies. High regression rate liquefying fuels developed in the late 1990s offer a potential solution to this problem.[8]
- Compared with liquid-based propulsion, re-fueling a partially or totally depleted hybrid rocket would present significant challenges, as the solid propellant cannot simply be pumped into a fuel tank. This may or may not be an issue, depending upon how the rocket is planned to be used.
In general, much less development work has been completed with hybrids than liquids or solids and it is likely that some of these disadvantages could be rectified through further investment in research and development.
One problem in designing large hybrid orbital rockets is that turbopumps become necessary to achieve high flow rates and pressurization of the oxidizer. This turbopump must be powered by something. In a traditional liquid-propellant rocket, the turbopump uses the same fuel and oxidizer as the rocket, since they are both liquid and can be fed to the pre-burner. But in a hybrid, the fuel is solid and cannot be fed to a turbopump's engine. Some hybrids use an oxidizer that can also be used as a monopropellant, such as nitromethane or hydrogen peroxide, and so a turbopump can run on it alone. But nitromethane and hydrogen peroxide are significantly less efficient than liquid oxygen, which cannot be used alone to run a turbopump. Another fuel would be needed, requiring its own tank and decreasing rocket performance.
الوقود
الاختيارات الشائعة
A reverse-hybrid rocket, which is not very common, is one where the engine uses a solid oxidizer and a liquid fuel. Some liquid fuel options are kerosene, hydrazine, and LH2. Common fuels for a typical hybrid rocket engine include polymers such as acrylics, polyethylene (PE), cross-linked rubber, such as HTPB, or liquefying fuels such as paraffin wax. Plexiglass was a common fuel, since the combustion could be visible through the transparent combustion chamber. Hydroxyl-terminated polybutadiene (HTPB) synthetic rubber is currently the most popular fuel for hybrid rocket engines, due to its energy, and due to how safe it is to handle. Tests have been performed in which HTPB was soaked in liquid oxygen, and it still did not become explosive. These fuels are generally not as dense as solid rocket motors, so they are often doped with aluminum to increase the density and therefore the rocket performance.[4]
. . . . . . . . . . . . . . . . . . . . . . . . . . . . . . . . . . . . . . . . . . . . . . . . . . . . . . . . . . . . . . . . . . . . . . . . . . . . . . . . . . . . . . . . . . . . . . . . . . . . . . . . . . . . . . . . . . . . . . . . . . . . . . . . . . . . . . . . . . . . . . . . . . . . . . . . . . . . . . . . . . . . . . . .
Grain manufacturing methods
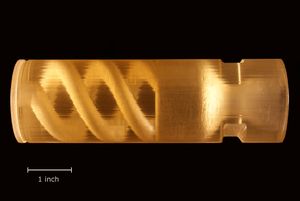
المؤكسد
الاختيارات الشائعة
Common oxidizers include gaseous or liquid oxygen, nitrous oxide, and hydrogen peroxide. For a reverse hybrid, oxidizers such as frozen oxygen and ammonium perchlorate are used.[4]
Proper oxidizer vaporization is important for the rocket to perform efficiently. Improper vaporization can lead to very large regression rate differences at the head end of the motor when compared to the aft end. One method is to use a hot gas generator to heat the oxidizer in a pre-combustion chamber. Another method is to use an oxidizer that can also be used as a monopropellant. A good example is hydrogen peroxide, which can be catalytically decomposed over a silver bed into hot oxygen and steam. A third method is to inject a propellant that is hypergolic with the oxidizer into the flow. Some of the oxidizer will decompose, heating up the rest of the oxidizer in the flow.[4]
سلامة الهجين
Generally, well designed and carefully constructed hybrids are very safe. The primary hazards associated with hybrids are:
- Pressure vessel failures – Chamber insulation failure may allow hot combustion gases near the chamber walls leading to a "burn-through" in which the vessel ruptures.
- Blow back – For oxidizers that decompose exothermically such as nitrous oxide or hydrogen peroxide, flame or hot gasses from the combustion chamber can propagate back through the injector, igniting the oxidizer and leading to a tank explosion. Blow-back requires gases to flow back through the injector due to insufficient pressure drop which can occur during periods of unstable combustion. Blow back is inherent to specific oxidizers and is not possible with oxidizers such as oxygen, or nitrogen tetroxide, unless fuel is present in the oxidizer tank.
- Hard starts – An excess of oxidizer in the combustion chamber prior to ignition, particularly for monopropellants such as nitrous oxide, can result in a temporary over-pressure or "spike" at ignition.
Because the fuel in a hybrid does not contain an oxidizer, it will not combust explosively on its own. For this reason, hybrids are classified as having no TNT equivalent explosive power. In contrast, solid rockets often have TNT equivalencies similar in magnitude to the mass of the propellant grain. Liquid-fuel rockets typically have a TNT equivalence calculated based on the amount of fuel and oxidizer which could realistically intimately combine before igniting explosively; this is often taken to be 10–20% of the total propellant mass. For hybrids, even filling the combustion chamber with oxidizer prior to ignition will not generally create an explosion with the solid fuel, the explosive equivalence is often quoted as 0%.
المنظمات العاملة
الشركات التجارية
الجامعات
الصواريخ عالية الطاقة
في الثقافة العامة
انظر أيضاً
المصادر
- ^ "GIRD-09". Encyclopedia Astronautix. Retrieved June 25, 2017.
- ^ "Hybrid rocket propulsion overview". Space Propulsion Group, Inc.
- ^ "A brief history of hybrid rocket technology". Space Propulsion Group, Inc. Archived from the original on July 16, 2011. Retrieved October 15, 2010.
- ^ أ ب ت ث ج ح خ د ذ ر ز س ش ص Humble, Ronald; Gary, Henry; Larson, Wiley (1995). Space Propulsion Analysis and Design. McGraw-Hill. ISBN 978-0-07-031320-0.
- ^ Shepherd, Shep (April 1954). "With the amateur – but serious – rocketeers out on the Mojave desert, it's Fourth of July the year around". Popular Mechanics. Hearst Magazines. pp. 81–85.
- ^ "This is how LMR and HPR got started ..." California Rocketry magazine.
- ^ قالب:Cite media see also Burt Rutan, Scaled Composites, SpaceShipOne.
- ^ "Wax Hybrids". Science@NASA. National Aeronautics and Space Administration (NASA). Archived from the original on May 23, 2009. Retrieved June 1, 2009.
قراءات إضافية
- Costa, Fernando de Souza; Vieira, Ricardo (2010). "Preliminary analysis of hybrid rockets for launching nanosats into LEO". Journal of the Brazilian Society of Mechanical Sciences and Engineering. 32 (4): 502–509. doi:10.1590/S1678-58782010000400012.
. . . . . . . . . . . . . . . . . . . . . . . . . . . . . . . . . . . . . . . . . . . . . . . . . . . . . . . . . . . . . . . . . . . . . . . . . . . . . . . . . . . . . . . . . . . . . . . . . . . . . . . . . . . . . . . . . . . . . . . . . . . . . . . . . . . . . . . . . . . . . . . . . . . . . . . . . . . . . . . . . . . . . . . .
وصلات خارجية
- "Developing and testing of a 2 kN hybrid rocket engine". hybrid-engine-development.de (in الألمانية).
- "Paraffin hybrid links". Portland State Aerospace Society. Archived from the original on 2006-09-01.
- "Hybridrocket". c-turbines.ch (private web page) (in الألمانية).