تمعدن حيوي
جزء من سلسلة عن |
التمعدن الحيوي |
---|
![]() |
التمعدن الحيوي Biomineralization هي إحدى طُرق التمعدن في علم الأحياء، وهي العملية التي تنتج فيها العضويات الحية المعادن [2] غالباً يكون هدفها تقسية وتصليب نسج موجودة. مثل هذه الأنسجة تسمّى بالنسج المتمعدنة وتعد ظاهرة واسعة الانتشار إلى حد كبير، تتضمن جميع الممالك التصنيفية أعداداً قادرة على تشكيل المعادن، وقد حُدد أكثر من 60 معدن مختلف في العضويات.[3][4][5] Examples include silicates in algae and diatoms, carbonates in invertebrates, and calcium phosphates and carbonates in vertebrates. These minerals often form structural features such as sea shells and the bone in mammals and birds.
Organisms have been producing mineralized skeletons for the past 550 million years. Calcium carbonates and calcium phosphates are usually crystalline, but silica organisms (sponges, diatoms...) are always non-crystalline minerals. Other examples include copper, iron, and gold deposits involving bacteria. Biologically formed minerals often have special uses such as magnetic sensors in magnetotactic bacteria (Fe3O4), gravity-sensing devices (CaCO3, CaSO4, BaSO4) and iron storage and mobilization (Fe2O3•H2O in the protein ferritin).
In terms of taxonomic distribution, the most common biominerals are the phosphate and carbonate salts of calcium that are used in conjunction with organic polymers such as collagen and chitin to give structural support to bones and shells.[6] The structures of these biocomposite materials are highly controlled from the nanometer to the macroscopic level, resulting in complex architectures that provide multifunctional properties. Because this range of control over mineral growth is desirable for materials engineering applications, there is interest in understanding and elucidating the mechanisms of biologically-controlled biomineralization.[7][8]
. . . . . . . . . . . . . . . . . . . . . . . . . . . . . . . . . . . . . . . . . . . . . . . . . . . . . . . . . . . . . . . . . . . . . . . . . . . . . . . . . . . . . . . . . . . . . . . . . . . . . . . . . . . . . . . . . . . . . . . . . . . . . . . . . . . . . . . . . . . . . . . . . . . . . . . . . . . . . . . . . . . . . . . .
الأحياء
If present on a super-cellular scale, biominerals are usually deposited by a dedicated organ, which is often defined very early in the embryological development. This organ will contain an organic matrix that facilitates and directs the deposition of crystals.[9] The matrix may be collagen, as in deuterostomes,[9] or based on chitin or other polysaccharides, as in molluscs.[10]
تشكل القواقع للمحاريات
The mollusc shell is a biogenic composite material that has been the subject of much interest in materials science because of its unusual properties and its model character for biomineralization. Molluscan shells consist of 95–99% calcium carbonate by weight, while an organic component makes up the remaining 1–5%. The resulting composite has a fracture toughness ~3000 times greater than that of the crystals themselves.[11] In the biomineralization of the mollusc shell, specialized proteins are responsible for directing crystal nucleation, phase, morphology, and growths dynamics and ultimately give the shell its remarkable mechanical strength. The application of biomimetic principles elucidated from mollusc shell assembly and structure may help in fabricating new composite materials with enhanced optical, electronic, or structural properties.
تمعدن العظام
تمعدن العظام هي عملية حيوية ترتبط بها معادن مثل الكالسيوم، الفوسفات، ومعادن أخرى لجعل العظام صلبة. حتى نهاية التسعينيات، اعتقد العلماء أن الاوستيوكالسين، پروتين تصنعه وتفرزه الخلايا العظمية، ويرتبط به الكالسيوم. وفي دراسة أُجريت بمختبر جامعة كلومبيا بنيويورك، عام 2019، على فئران مهندسة وراثياً لا تحتوي على الاوستيوكالسين، كان من المتوقع أن تصبح عظام هذه الفئران هشة، لكن لمن يحدث هذا، فقط حدثت عملية تمعدن العظام بشكل طبيعي تماماً، دون أن يكون للاوستيوكالسين أي تأثير على هذه العملية. وفي الواقع، كانت عظام تلك الفئران أكثر كثافة عن ذي قبل. وعند فحص العظام، تطلب الأمر من الباحثين حفر المزيد من الأنسجة الدهنية، مما يقترح أن غياب الاوستيوكالسين قد أدى إلى تراكم الدهون في أنسجة الفئران.[12] وكان تفسير العلماء أن عند نزع الاوستيوكالسين من العظام انتقل إلى الدم، ومن ثم إلى الأنسجة الدهنية، حيث أثر بطريقة ما على نظام التمثيل الغذائي للفئران، ولعب دوراً في تراكم الدهونأي أن الاوستيوكالسين ليس مجرد پروتين، بل قد يكون هرمون له دور في عملية التمثيل الغذائي. مما يعني العظام مسئولة عن إفراز هرمونات ضرورية لصحة الحيوان، وبهذه النتيجة انضم الهيكل العظمي إلى مجموعة الأنسجة المشاركة مع الغدد الصماء، بالترادف مع الجهاز العصبي لإفراز الهرمونات المنظمة للعمليات الحيوية في الجسم.
انتاج وتحلل المعادن في الفطريات
الكيمياء
Because extracellular[13] iron is strongly involved in inducing calcification,[14][15] its control is essential in developing shells; the protein ferritin plays an important role in controlling the distribution of iron.[16] The most common mineral present in biomineralization is hydroxyapatite (HA), which is a naturally occurring mineral form of calcium apatite with the formula Ca10(PO4)6(OH)2. Hydroxyapatite crystals are found in many biological materials including bones,[17] fish scales,[18] and cartilage.[19] Each material has a mineral content which corresponds with the required mechanical properties, where increasing HA content typically leads to increased stiffness but reduced extensibility.[20]
التطور
The first evidence of biomineralization dates to some [21][22] and sponge-grade organisms may have formed calcite skeletons .[23] But in most lineages, biomineralization first occurred in the Cambrian or Ordovician periods.[24] Organisms used whichever form of calcium carbonate was more stable in the water column at the point in time when they became biomineralized,[25] and stuck with that form for the remainder of their biological history[26] (but see [27] for a more detailed analysis). The stability is dependent on the Ca/Mg ratio of seawater, which is thought to be controlled primarily by the rate of sea floor spreading, although atmospheric CO2 levels may also play a role.[25]
,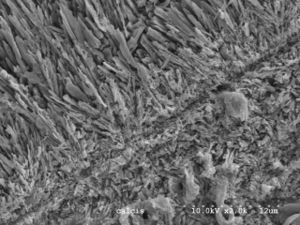
Type of mineralization | Examples of organisms |
---|---|
Calcium carbonate (calcite or aragonite) | |
Silica |
|
Apatite (phosphate carbonate) |
|
علم الأحياء الفلكي
It has been suggested that biominerals could be important indicators of extraterrestrial life and thus could play an important role in the search for past or present life on Mars. Furthermore, organic components (biosignatures) that are often associated with biominerals are believed to play crucial roles in both pre-biotic and biotic reactions.[28]
On January 24, 2014, NASA reported that current studies by the Curiosity and Opportunity rovers on the planet Mars will now be searching for evidence of ancient life, including a biosphere based on autotrophic, chemotrophic and/or chemolithoautotrophic microorganisms, as well as ancient water, including fluvio-lacustrine environments (plains related to ancient rivers or lakes) that may have been habitable.[29][30][31][32] The search for evidence of habitability, taphonomy (related to fossils), and organic carbon on the planet Mars is now a primary NASA objective.[29][30]
قائمة المعادن
Examples of biogenic minerals include:[33]
- Apatite in bones and teeth.
- Aragonite, calcite, fluorite in vestibular systems (part of the inner ear) of vertebrates.
- Aragonite and calcite in travertine and biogenic silica (siliceous sinter, opal) deposited through algal action.
- Hydroxylapatite formed by mitochondria.
- Magnetite and greigite formed by magnetotactic bacteria.
- Pyrite and marcasite in sedimentary rocks deposited by sulfate-reducing bacteria.
- Quartz and diamonds formed from bacterial action on fossil fuels (gas, oil, coal).
الاستخدامات المحتملة
Most traditional approaches to synthesis of nanoscale materials are energy inefficient, requiring stringent conditions (e.g., high temperature, pressure or pH) and often produce toxic byproducts. Furthermore, the quantities produced are small, and the resultant material is usually irreproducible because of the difficulties in controlling agglomeration.[34] In contrast, materials produced by organisms have properties that usually surpass those of analogous synthetically manufactured materials with similar phase composition. Biological materials are assembled in aqueous environments under mild conditions by using macromolecules. Organic macromolecules collect and transport raw materials and assemble these substrates and into short- and long-range ordered composites with consistency and uniformity. The aim of biomimetics is to mimic the natural way of producing minerals such as apatites. Many man-made crystals require elevated temperatures and strong chemical solutions, whereas the organisms have long been able to lay down elaborate mineral structures at ambient temperatures. Often, the mineral phases are not pure but are made as composites that entail an organic part, often protein, which takes part in and controls the biomineralisation. These composites are often not only as hard as the pure mineral but also tougher, as the micro-environment controls biomineralisation.
. . . . . . . . . . . . . . . . . . . . . . . . . . . . . . . . . . . . . . . . . . . . . . . . . . . . . . . . . . . . . . . . . . . . . . . . . . . . . . . . . . . . . . . . . . . . . . . . . . . . . . . . . . . . . . . . . . . . . . . . . . . . . . . . . . . . . . . . . . . . . . . . . . . . . . . . . . . . . . . . . . . . . . . .
ملوثات اليورانيوم في المياه الجوفية
Biomineralization may be used to remediate groundwater contaminated with uranium.[35] The biomineralization of uranium primarily involves the precipitation of uranium phosphate minerals associated with the release of phosphate by microorganisms. Negatively charged ligands at the surface of the cells attract the positively charged uranyl ion (UO22+). If the concentrations of phosphate and UO22+ are sufficiently high, minerals such as autunite (Ca(UO2)2(PO4)2•10-12H2O) or polycrystalline HUO2PO4 may form thus reducing the mobility of UO22+. Compared to the direct addition of inorganic phosphate to contaminated groundwater, biomineralization has the advantage that the ligands produced by microbes will target uranium compounds more specifically rather than react actively with all aqueous metals. Stimulating bacterial phosphatase activity to liberate phosphate under controlled conditions limits the rate of bacterial hydrolysis of organophosphate and the release of phosphate to the system, thus avoiding clogging of the injection location with metal phosphate minerals.[35] The high concentration of ligands near the cell surface also provides nucleation foci for precipitation, which leads to higher efficiency than chemical precipitation.[36]
انظر أيضاً
مصادر
- ^ Vert M, Doi Y, Hellwich KH, Hess M, Hodge P, Kubisa P, Rinaudo M, Schué F (11 January 2012). "Terminology for biorelated polymers and applications (IUPAC Recommendations 2012)". Pure and Applied Chemistry. 84 (2): 377–410. doi:10.1351/PAC-REC-10-12-04. S2CID 98107080.
- ^ Harris, Ph.D., Edward D. Minerals in Food Nutrition, Metabolism, Bioactivity. Lancaster, PA: DEStech Publications, Inc. p. 378.
{{cite book}}
: Cite has empty unknown parameters:|lay-date=
,|subscription=
,|nopp=
,|last-author-amp=
,|name-list-format=
,|lay-source=
,|registration=
, and|lay-summary=
(help) - ^ Sigel A, Sigel H, Sigel RK, eds. (2008). Biomineralization: From Nature to Application. Metal Ions in Life Sciences. Vol. 4. Wiley. ISBN 978-0-470-03525-2.
- ^ Weiner S, Lowenstam HA (1989). On biomineralization. Oxford [Oxfordshire]: Oxford University Press. ISBN 978-0-19-504977-0.
- ^ Cuif JP, Dauphin Y, Sorauf JE (2011). Biominerals and fossils through time. Cambridge. ISBN 978-0-521-87473-1.
- ^ Vinn O (2013). "Occurrence, formation and function of organic sheets in the mineral tube structures of Serpulidae (polychaeta, Annelida)". PLOS ONE. 8 (10): e75330. Bibcode:2013PLoSO...875330V. doi:10.1371/journal.pone.0075330. PMC 3792063. PMID 24116035.
- ^ Boskey AL (1998). "Biomineralization: conflicts, challenges, and opportunities". Journal of Cellular Biochemistry. 30–31 (S30-31): 83–91. doi:10.1002/(SICI)1097-4644(1998)72:30/31+<83::AID-JCB12>3.0.CO;2-F. PMID 9893259. S2CID 46004807.
- ^ Sarikaya M (December 1999). "Biomimetics: materials fabrication through biology". Proceedings of the National Academy of Sciences of the United States of America. 96 (25): 14183–14185. Bibcode:1999PNAS...9614183S. doi:10.1073/pnas.96.25.14183. PMC 33939. PMID 10588672.
- ^ أ ب خطأ استشهاد: وسم
<ref>
غير صحيح؛ لا نص تم توفيره للمراجع المسماةLivingston2006
- ^ Checa, A.; Ramírez-Rico, J.; González-Segura, A.; Sánchez-Navas, A. (2009). "Nacre and false nacre (foliated aragonite) in extant monoplacophorans (=Tryblidiida: Mollusca)". Die Naturwissenschaften. 96 (1): 111–122. Bibcode:2009NW.....96..111C. doi:10.1007/s00114-008-0461-1. PMID 18843476.
- ^ Currey, J. D. (1999). "The design of mineralised hard tissues for their mechanical functions". The Journal of Experimental Biology. 202 (Pt 23): 3285–3294. PMID 10562511.
- ^ "Hormones". aeon.co. 2019-02-19. Retrieved 2019-02-21.
- ^ Gabbiani G, Tuchweber B (1963). "The role of iron in the mechanism of experimental calcification". J Histochem Cytochem. 11 (6): 799–803. doi:10.1177/11.6.799.
- ^ Schulz, K.; Zondervan, I.; Gerringa, L.; Timmermans, K.; Veldhuis, M.; Riebesell, U. (2004). "Effect of trace metal availability on coccolithophorid calcification". Nature. 430 (7000): 673–676. Bibcode:2004Natur.430..673S. doi:10.1038/nature02631. PMID 15295599.
- ^ Anghileri, L. J.; Maincent, P.; Cordova-Martinez, A. (1993). "On the mechanism of soft tissue calcification induced by complexed iron". Experimental and Toxicologic Pathology. 45 (5–6): 365–368. doi:10.1016/S0940-2993(11)80429-X. PMID 8312724.
- ^ Jackson, D. J.; Wörheide, G.; Degnan, B. M. (2007). "Dynamic expression of ancient and novel molluscan shell genes during ecological transitions". BMC Evolutionary Biology. 7: 160. doi:10.1186/1471-2148-7-160. PMC 2034539. PMID 17845714.
{{cite journal}}
: CS1 maint: unflagged free DOI (link) - ^ Roy, Della M. (1974). "Hydroxyapatite formed from coral skeletal carbonate by hydrothermal exchange". Nature. 247: 220–222. doi:10.1038/247220a0.
- ^ Onozato, Hiroshi (1979). "Studies on fish scale formation and resorption". Cell and Tissue Research. 201 (3): 409–422. doi:10.1007/BF00236999.
- ^ Ohirta, T (1986). "Hydroxyapatite deposition in articular cartilage by intra-articular injections of methylprednisolone. A histological, ultrastructural, and x-ray-microprobe analysis in rabbits". The Journal of Bone and Joint Surgery. 68 (4): 509–520.
- ^ Sherman, Vincent R. (2008). "The materials science of collagen". Journal of the Mechanical Behavior of Biomedical Materials. 61 (5): 529–534. doi:10.1016/j.bjps.2007.06.004. PMID 17652049.
- ^ Porter, S. (2011). "The rise of predators". Geology. 39 (6): 607–608. Bibcode:2011Geo....39..607P. doi:10.1130/focus062011.1.
- ^ Cohen, P. A.; Schopf, J. W.; Butterfield, N. J.; Kudryavtsev, A. B.; MacDonald, F. A. (2011). "Phosphate biomineralization in mid-Neoproterozoic protists". Geology. 39: 539–542. Bibcode:2011Geo....39..539C. doi:10.1130/G31833.1.
- ^ Maloof, A. C.; Rose, C. V.; Beach, R.; Samuels, B. M.; Calmet, C. C.; Erwin, D. H.; Poirier, G. R.; Yao, N.; Simons, F. J. (2010). "Possible animal-body fossils in pre-Marinoan limestones from South Australia". Nature Geoscience. 3 (9): 653–659. Bibcode:2010NatGe...3..653M. doi:10.1038/ngeo934.
- ^ Wood, R. A. (2002). "Proterozoic Modular Biomineralized Metazoan from the Nama Group, Namibia". Science. 296 (5577): 2383–2386. Bibcode:2002Sci...296.2383W. doi:10.1126/science.1071599. ISSN 0036-8075. PMID 12089440.
- ^ أ ب Zhuravlev, A. Y.; Wood, R. A. (2008). "Eve of biomineralization: Controls on skeletal mineralogy" (PDF). Geology. 36 (12): 923. Bibcode:2008Geo....36..923Z. doi:10.1130/G25094A.1.
- ^ Porter, S. M. (Jun 2007). "Seawater chemistry and early carbonate biomineralization". Science. 316 (5829): 1302. Bibcode:2007Sci...316.1302P. doi:10.1126/science.1137284. ISSN 0036-8075. PMID 17540895.
- ^ Maloof, A. C.; Porter, S. M.; Moore, J. L.; Dudas, F. O.; Bowring, S. A.; Higgins, J. A.; Fike, D. A.; Eddy, M. P. (2010). "The earliest Cambrian record of animals and ocean geochemical change". Geological Society of America Bulletin. 122 (11–12): 1731–1774. Bibcode:2010GSAB..122.1731M. doi:10.1130/B30346.1.
- ^ Steele, Andrew; Beaty, David, eds. (September 26, 2006). "Final report of the MEPAG Astrobiology Field Laboratory Science Steering Group (AFL-SSG)" (.doc). The Astrobiology Field Laboratory. U.S.A.: Mars Exploration Program Analysis Group (MEPAG) - NASA. p. 72. Retrieved 2009-07-22.
{{cite book}}
: Cite uses deprecated parameter|authors=
(help) - ^ أ ب Grotzinger, John P. (January 24, 2014). "Introduction to Special Issue - Habitability, Taphonomy, and the Search for Organic Carbon on Mars". Science. 343 (6169): 386–387. Bibcode:2014Sci...343..386G. doi:10.1126/science.1249944. PMID 24458635. Retrieved January 24, 2014.
- ^ أ ب "Special Issue - Table of Contents - Exploring Martian Habitability". Science. 343 (6169): 345–452. January 24, 2014. Retrieved January 24, 2014.
{{cite journal}}
: Cite uses deprecated parameter|authors=
(help) - ^ "Special Collection - Curiosity - Exploring Martian Habitability". Science. January 24, 2014. Retrieved January 24, 2014.
{{cite journal}}
: Cite uses deprecated parameter|authors=
(help) - ^ Grotzinger, J.P.; et al. (January 24, 2014). "A Habitable Fluvio-Lacustrine Environment at Yellowknife Bay, Gale Crater, Mars". Science. 343 (6169): 1242777. Bibcode:2014Sci...343A.386G. doi:10.1126/science.1242777. PMID 24324272. Retrieved January 24, 2014.
- ^ Corliss, William R. (Nov–Dec 1989). "Biogenic Minerals". Science Frontiers. 66.
{{cite journal}}
: CS1 maint: date format (link) - ^ Thomas, George Brinton; Komarneni, Sridhar; Parker, John (1993). Nanophase and Nanocomposite Materials: Symposium Held December 1–3, 1992, Boston, Massachusetts, U.S.A. (Materials Research Society Symposium Proceedings). Pittsburgh, Pa: Materials Research Society. ISBN 1-55899-181-6.
- ^ أ ب Newsome, L.; Morris, K.; Lloyd, J. R. (2014). "The biogeochemistry and bioremediation of uranium and other priority radionuclides". Chemical Geology. 363: 164–184. doi:10.1016/j.chemgeo.2013.10.034.
{{cite journal}}
: Unknown parameter|last-author-amp=
ignored (|name-list-style=
suggested) (help) - ^ Lloyd, J. R.; Macaskie, L. E (2000). Environmental microbe-metal interactions: Bioremediation of radionuclide-containing wastewaters. Washington, DC: ASM Press. pp. 277–327. ISBN 1-55581-195-7.
{{cite book}}
: Unknown parameter|last-author-amp=
ignored (|name-list-style=
suggested) (help)
وصلات خارجية
- An overview of the bacteria involved in biomineralization from the Science Creative Quarterly
- ‘Data and literature on modern and fossil Biominerals’: http://biomineralisation.blogspot.fr
- Minerals and the Origins of Life (Robert Hazen, NASA) (video, 60m, April 2014).
- Biomineralization web-book: bio-mineral.org
- Special German Research Project About the Principles of Biomineralization